David L. Rimoin
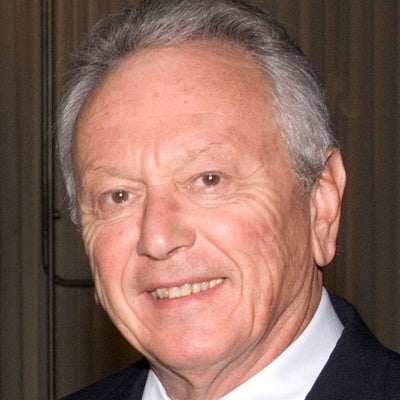
David L. Rimoin, Steven Spielberg Chair and Director of the Medical Genetics Institute at Cedars-Sinai Medical Center in Los Angeles, was best known for his work on the genetics of skeletal dysplasia, and for his pioneering recognition in 1967 that common diseases like diabetes mellitus were not single-gene phenotypes, but could result from any one of a group of genetic variations. Dr. Rimoin died in May, 2012.
Biographical Sketch
David Rimoin was born in 1936 in Montreal, Canada. He earned his MD from McGill University in 1961, and completed residency training there and at Johns Hopkins, where he received a PhD in human genetics in 1967. He worked at Washington University in St. Louis for three years before coming to Los Angeles in 1970, where he built divisions of human genetics first at Harbor-UCLA and then, from 1986, at Cedars-Sinai Medical Center, also affiliated with UCLA. Currently, Dr. Rimoin is Professor of Pediatrics and Director of the Medical Genetics Institute at Cedars-Sinai. In addition to his institutional leadership, Dr. Rimoin is perhaps best known for his pioneering demonstration in 1970 that diabetes mellitus was the phenotypic reflection of not a single genetic allele, but of multiple possible genetic variants; this work laid the foundation for the field of common disease genetics. He is also recognized for his pioneering studies of genetic syndromes of dwarfism and skeletal dysplasia, and for his classic co-authored textbook, Emery and Rimoin’s Principles and Practice of Medical Genetics, which first appeared in 1983. Dr. Rimoin died in 2012.
Timeline
1936 | Born November 9, in Montreal, Quebec, Canada |
1957 | Graduated from McGill University with First Class Honors in Genetics |
1961 | Received M.D. and M.Sc. degrees from McGill |
1961-64 | Internship and Residencies in Montreal and Baltimore |
1966 | Reported isolation of human growth deficiency hormone gene |
1967 | Published paper on genetic heterogeneity of diabetes mellitus |
1967 | Earned Ph.D. in Human Genetics from Johns Hopkins University |
1967-70 | Director, Medical Genetics Clinic, and Assistant Professor of Medicine and Pediatrics, Washington University St. Louis |
1967-70 | RCDA from NICHD; first grant on skeletal dysplasias (renewed for >30 years) |
1969 | Paper on subresponsiveness to human growth hormone in African pygmies |
1970-73 | Associate Professor of Pediatrics & Medicine, UCLA School of Medicine |
1970-86 | Chief, Division of Medical Genetics, Harbor-UCLA Medical Center |
1973 | Professor of Pediatrics and Medicine, UCLA |
1976 | Ross Outstanding Young Investigator Award, Western Soc for Pediatric Research Mead Johnson Aware for Research in Pediatrics, American Acad of Pediatrics |
1979-83 | Founding President, American Board of Medical Genetics |
1983 | First edition of Emery and Rimoin’s Principles and Practice of Medical Genetics |
1984 | President, American Society of Human Genetics |
1986-2004 | Chairman, Department of Pediatrics and Director of Medical Genetics – Birth Defects Center, Cedars-Sinai Medical Center, Los Angeles |
1992-98 | Founding President, American College of Medical Genetics |
1993 | President, Council of Medical Genetics Organizations |
1997 | March of Dimes Lifetime Achievement in the Genetics Sciences Award |
2004 | Director, Medical Genetics Institute, Cedars-Sinai Medical Center |
Topical Outline
The interview is organized chronologically, beginning with Rimoin’s early education and student research in genetics; family background; residency in Montreal and introduction to biochemical genetics; invitation to Johns Hopkins residency, move to Baltimore, and genetics research at Hopkins; interest in genetics of endocrine disorders, including diabetes and growth hormone deficiency; studies of genetics in isolated populations; first book, job at Washington University, and first RCDA; transition to pediatrics; move to Los Angeles and building a new division at Harbor-UCLA and then at Cedars-Sinai; work with the American Board and American College of Medical Genetics; structuring relationships with genetic counselors; marriages and family. Major topics of interest include the state of the field of medical genetics in the 1950s and 1960s; genetics of endocrine disorders; Hopkins experience and origins of Mendelian Inheritance in Man; possibilities of genetic disease prevention; diabetes as a model for common disease genetics; tracing the genetic mechanisms of disease; Emery and Rimoin’s Principles and Practices; experiences with treating patients with skeletal dysplasias and with the Little People’s advocacy group; future possibilities of gene therapy; and reminiscences of Victor McKusick, Luca Cavalli-Sforza, and Michael Kaback
DAVID RIMOIN: A SELECTION OF HIS MAJOR PAPERS
- Rimoin DL, Merimee TJ, McKusick VA: Growth hormone deficiency in man: an isolated, recessively inherited defect. Science 152: 1635-1637, 1966.http://www.sciencemag.org/cgi/reprint/152/3729/1635
- Rimoin DL: Genetics of diabetes mellitus. Diabetes. 16: 346-351, 1967. Not available online. Abstract: The genetics of diabetes mellitus is a confused subject. There is good evidence that genetic factors are important in the etiology of diabetes, but little is known about the mode of genetic transmission. Conclusive studies will not be possible until the basic defect in diabetes is elucidated and a reliable marker for prediabetes obtained. The hypothesis that diabetes mellitus represents a genetically heterogeneous group of disorders is discussed.
- Rimoin DL, Merimee TJ, Rabinowtiz D, McKusick VA, Cavalli-Sforza L: Growth hormone in the African pygmies. Lancet. 2: 523-526, 1967. Not available online.
- Rimoin DL, Merimee TJ, Rabinowitz D, Cavalli-Sforza LL, McKusick VA: Peripheral subresponsiveness to human growth hormone in the African pygmies. New England Journal of Medicine 218:1383-1388, 1969. Not available online.
- Rimoin DL, Hughes GN, Kaufman RL, Rosenthal RE, McAlister WH, Silberberg R: Endochondral ossification in achondroplastic dwarfism. New England Journal of Medicine 283:728-735, 1970. Not available online. Abstract: The literature concerning endochondral histopathology in the chondrodystrophies is misleading because the true heterogeneity of this group of disorders was not recognized until relatively recently. Accordingly, biopsies of the chondro-osseous junctions of rib and iliac crest were performed on seven typical achondroplastic dwarfs ranging in age from 14 months to 35 years. In contrast to previous reports, histologic studies of this biopsy material demonstrate regular, well organized endochrondral ossification in classic achondroplasia. These observations suggest that the basic defect in achondroplasia may be a quantitative decrease in the rate of endochondral ossification which, in conjunction with undisturbed periosteal bone formation, results in the short, squat shape of the tubular bones. These findings necessitate a reclassification of the many animal chondrodystrophic mutants, some of which have been mislabeled as models of achrondroplasia because of their abnormal endochrondral histopathology.
- Sly WS, Quinton BA, McAlister WH, Rimoin DL: Beta glucuronidase deficiency: report of clinical, radiologic, and biochemical features of a new mucopolysaccharidosis. Journal of Pediatrics 84:249-257, 1973. Not available online.
- Rimoin DL, Hollister DW, Lachman RS, Kaufman RL, McAlister WH, Rosenthal RE, Hughes GNF: Histological studies in the chondrodystrophies. Birth Defects: Original Article Series, 10(12):274-295, 1974. Not available online.
- Rimoin DL: The chondrodystrophies: In: Advances in Human Genetics, Fifth Volume, Harris, H. and Hirschhorn, K., editors, Plenum Publishing Corporation, pp. 1-118, 1975. Not available online.
- Sillence DO, Rimoin DL: Classification of osteogenesis imperfecta. Lancet 1:1041-1042, 1978. Not available online.
- Sillence DO, Horton WA, Rimoin DL: Morphologic studies in the skeletal dysplasias. American Journal of Pathology 96:813-870, 1979. Not available online. Abstract: Considerable progress has been made in the delineation of the genetic skeletal dysplasias, a heterogeneous group of disorders, that consist of over 80 distinct conditions. Morphologic studies have added a further dimension to the delineation of these conditions, their diagnosis, and the investigation of their pathogenetic mechanisms. In certain diseases, the morphologic alterations are characteristic and pathognomonic. In others only nonspecific alterations are observed, whereas in still other disorders growth-plate structure is essentially normal. Histologic, histochemical, and electronmicroscopic studies of growth-plate cartilage have provided new insights into the complexity of morphogenetic events in normal growth through the demonstration of morphologic defects in the genetic disorders of skeletal growth. As yet, very little is known of the biochemical abnormalities underlying the morphologic abnormalities. However, the great variety of morphologic findings points to a number of different pathogenetic defects in the synthesis, release, and assembly of connective tissue macromolecules and in the cells involved in growth-plate metabolism.
- Rotter JI, Anderson CE, Rubin R, Congleton JE, Teraskai PI, Rimoin DL: An HLA genotype study of IDDM: heterogeneity and the excess of HLA DR3/DR4 heterozygotes. Diabetes 32:169-174, 1983. Not available online. Abstract: The genetics of insulin-dependent diabetes mellitus (IDDM) is currently an area of controversy, with some investigators proposing heterogeneity within the HLA region and even the existence of non-HLA-linked susceptibility genes, and others maintaining that a simple autosomal recessive gene linked to HLA with reduced penetrance is an adequate explanation. To resolve this latter question, we report here a simple method of testing whether a single HLA-linked susceptibility gene is inherited in a recessive fashion when it is associated with two different HLA alleles, as is the case for IDDM. It is shown that if the number of DR3/DR4 heterozygotes in a diabetic population exceeds the combined sum of DR3/3 and DR4/4 homozygotes in that same diabetic population, then a recessive mode of inheritance can be rejected. The advantages of the method are that it does not depend on ratios, as do relative risk calculations, nor does it depend on control data, but is based only on studies of the diabetics themselves. With data on 193 genotyped IDDM patients, we can clearly reject the recessive mode of inheritance, since the number of heterozygotes is 68 compared with a maximum of 22 homozygotes (P less than 10(-4)). Eight other published studies are in concordance with these results. Therefore, a nonparametric test, independent of the significance of any individual study, rejects equality of heterozygotes and homozygotes (P less than 0.002) and rejects the simple recessive mode of inheritance as a direct consequence. We conclude that more complex modes of inheritance of HLA-linked IDDM susceptibility, such as two different diabetogenic alleles or multiple loci, must be entertained.
- Lee B, Vissing H, Ramirez F, Rogers D, Rimoin DL: Identification of the molecular defect in a family with spondyloepiphyseal dysplasia. Science, 244: 978-980, 1989. http://www.sciencemag.org/cgi/reprint/244/4907/978. Abstract: Spondyloepiphyseal dysplasias (SED) are a heterogeneous group of inherited disorders characterized by disproportionate short stature and pleiotropic involvement of the skeletal and ocular systems. Evidence has suggested that SED may result from structural defects in type II collagen. To confirm the validity of this hypothesis, the structure of the “candidate” type II collagen gene (COL2A1) has been directly examined in a relatively large SED family. Coarse scanning of the gene by Southern blot hybridization identified an abnormal restriction pattern in one of the affected members of the kindred. Analysis of selected genomic fragments, amplified by the polymerase chain reaction, precisely localized the molecular defect and demonstrated that all affected family members carried the same heterozygous single-exon deletion. As a consequence of the mutation, nearly 90 percent of the assembled type II collagen homotrimers are expected to contain one or more procollagen subunits harboring an interstitial deletion of 36 amino acids in the triple helical domain.
- Tavormina PL, Shiang R, Thompson LM, Ya-Zhen Z, Wilkin DJ, Lachman RS, Wilcox WR, Rimoin, DL, Cohn DH and Wasmuth JJ: Mutations affecting distinct domains of FRFR3 cause different types of thanatophoric dysplasia. Nature Genetics 9:321-328,1995. http://www.nature.com/ng/journal/v9/n3/abs/ng0395-321.html. Abstract: Thanatophoric dysplasia (TD), the most common neonatal lethal skeletal dysplasia, affects one out of 20,000 live births. Affected individuals display features similar to those seen in homozygous achondroplasia. Mutations causing achondroplasia are in FGFR3, suggesting that mutations in this gene may cause TD. A sporadic mutation causing a Lys650Glu change in the tyrosine kinase domain of FGFR3 was found in 16 of 16 individuals with one type of TD. Of 39 individuals with a second type of TD, 22 had a mutation causing an Arg248Cys change and one had a Ser371Cys substitution, both in the extracellular region of the protein. None of these mutations were found in 50 controls showing that mutations affecting different functional domains of FGFR3 cause different forms of this lethal disorder.
- Briggs, MD, Hoffman, SMG, King LM, Olsen AS, Mohrenweiser H, Leroy JG, Mortier, GR, Rimoin DL, Lachman RS, Gaines ES, Cekleniak JA, Knowlton RG and Cohn DH: Pseudoachondroplasia and multiple epiphyseal dysplasia produced by mutations in the calcium binding domain of cartilage oligomeric matrix protein (COMP). Nature Genetics 10:330-336,1995. http://www.nature.com/ng/journal/v10/n3/abs/ng0795-330.html. Abstract: Pseudoachondroplasia (PSACH) and multiple epiphyseal dysplasia (MED) are dominantly inherited chondrodysplasias characterized by short stature and early-onset osteoarthrosis. The disease genes in families with PSACH and MED have been localized to an 800 kilobase interval on the short arm of chromosome 19. Recently the gene for cartilage oligomeric matrix protein (COMP) was localized to chromosome 19p13.1. In three patients with these diseases, we identified COMP mutations in a region of the gene that encodes a Ca++ binding motif. Our data demonstrate that PSACH and some forms of MED are allelic and suggest an essential role for Ca++ binding in COMP structure and function.
- Superti-Furga A, Bonafe L, Rimoin DL: Molecular-Pathogenetic Classification of Genetic Disorders of the Skeleton. Am. J. Med Genetics, 106: 282-293, 2001. http://www3.interscience.wiley.com/cgi-bin/abstract/89016193. Abstract: Genetic disorders of the skeleton (skeletal dysplasias and dysostoses) are a large and disparate group of diseases whose unifying features are malformation, disproportionate growth, and deformation of the skeleton or of individual bones or groups of bones. To cope with the large number of different disorders, the “Nosology and Classification of the Osteochondrodysplasias,” based on clinical and radiographic features, has been designed and revised periodically. Biochemical and molecular features have been partially implemented in the Nosology, but the rapid accumulation of knowledge on genes and proteins cannot be easily merged into the clinical-radiographic classification. We present here, as a complement to the existing Nosology, a classification of genetic disorders of the skeleton based on the structure and function of the causative genes and proteins. This molecular-pathogenetic classification should be helpful in recognizing metabolic and signaling pathways relevant to skeletal development, in pointing out candidate genes and possible therapeutic targets, and more generally in bringing the clinic closer to the basic science laboratory and in promoting research in this field.
- Cohn DH, Ehtesham N, Krakow D, Unger S, Shanske A, Reinker K, Powell BR, Rimoin DL: Mental Retardation and Abnormal Skeletal Development (Dyggve-Melchior-Clausen Dysplasia) due to Mutations in a Novel, Evolutionarily Conserved Gene. Am. J. Hum. Genet.72:419-428,2003. http://www.pubmedcentral.nih.gov/articlerender.fcgi?tool=pubmed &pubmedid=12491225. Abstract: Dyggve-Melchior-Clausen dysplasia (DMC) and Smith-McCort dysplasia (SMC) are similar, rare autosomal recessive osteochondrodysplasias. The radiographic features and cartilage histology in DMC and SMC are identical. However, patients with DMC exhibit significant developmental delay and mental retardation, the major features that distinguish the two conditions. Linkage studies localized the SMC and DMC disease genes to chromosome 18q12-21.1, providing evidence suggesting that they are allelic disorders. Sequence analysis of the coding exons of the FLJ90130 gene, a highly evolutionarily conserved gene within the recombination interval defined in the linkage study, identified mutations in SMC and DMC patients. The affected individuals in two consanguinous DMC families were homozygous for a stop codon mutation and a frameshift mutation, respectively, demonstrating that DMC represents the FLJ90130-null phenotype. The data confirm the hypothesis that SMC and DMC are allelic disorders and identify a gene necessary for normal skeletal development and brain function.
- Krakow D, Robertson SP, King LM, Morgan T, Sebald ET, Bertolotto C, Wachsmann-Hogiu S, Acuna D, Shapiro SS, Takafuta T, Aftimos S, Kim CA, Firth H, Steiner CE, Cormier-Daire V, Superti-Furga A, Bonafe L, Graham JM, Grix A, Bacino CA, Allanson J, Bialer MG, Lachman RS, Rimoin DL, Cohn DH. Mutations in the gene encoding filamin B disrupt vertebral segmentation, joint formation and skeletogenesis. Nature Genetics 36: 405-410, 2004. http://www.nature.com/ng/journal/v36/n4/abs/ng1319.html. Abstract: The filamins are cytoplasmic proteins that regulate the structure and activity of the cytoskeleton by cross-linking actin into three-dimensional networks, linking the cell membrane to the cytoskeleton and serving as scaffolds on which intracellular signaling and protein trafficking pathways are organized (reviewed in refs. 1,2). We identified mutations in the gene encoding filamin B in four human skeletal disorders. We found homozygosity or compound heterozygosity with respect to stop-codon mutations in autosomal recessive spondylocarpotarsal syndrome (SCT, OMIM 272460) and missense mutations in individuals with autosomal dominant Larsen syndrome (OMIM 150250) and the perinatal lethal atelosteogenesis I and III phenotypes (AOI, OMIM 108720; AOIII, OMIM 108721). We found that filamin B is expressed in human growth plate chondrocytes and in the developing vertebral bodies in the mouse. These data indicate an unexpected role in vertebral segmentation, joint formation and endochondral ossification for this ubiquitously expressed cytoskeletal protein.
1. The Early Years in Montreal and Residency at McGill
MARCIA MELDRUM: Good morning Dr. Rimoin. We’re here in Dr. David Rimoin’s office at Cedars-Sinai. It’s January 23, 2002, and it’s 9:20. We’re starting this interview for the History of Medical Genetics project. I’d like to go back and start with your origins in Montreal. You grew up in Montreal, is that right?
DAVID RIMOIN: Yes. I grew up in Montreal. I was born in Montreal and lived there until after a couple years of my medical residency. My parents were both born in Canada and their parents came from Russia and Rumania. So I lived in Montreal, went to grade school there, high school, then went to McGill University for a bachelor of science degree. During that time, actually, in my first year of college as a sixteen-year-old student, I had to decide how I was going to get into medical school. Genetics was a new honors program that had just been started at McGill, so at age seventeen, as a sophomore in college, I started an honors genetics program at McGill and formed a close relationship with F. Clark Fraser [1], whom I started working for on a part-time basis.
My actual first job in genetics, aside from filing reprints and slides, was to get a job in his mouse lab, where he was doing studies on the effects of teratogens[2], especially cortisone, on cleft palate mice. He had to time the pregnancies very carefully, so my first job was to go into the mouse room every morning and check hundreds of mice for vaginal plugs to see if we could find out if they had been impregnated the night before. I always thought this was something that should go on What’s My Line? [3] It probably explains why I never went into ob/gyn.
So I did this program, and as I was going through it and taking courses with a variety of co students, my co student and classmate was Dorothy Warburton [4] at that time. Leonard Pinsky [5] was a year ahead of me. We really had a very excellent group of people and it was an exciting time taking, really, a general biology course and a course in medical and basic genetics.
When it came time to finish my bachelor’s degree, they wanted me to pursue a Ph.D. in human genetics, or basic genetics, at that time. I was determined to go to medical school and finally got in, so we created a master’s program, which I did over the summers of medical school. Not being enthralled with working with mice at that time, I elected to work with Julius and Kay Metrakos [6], who were doing their pioneering studies in the genetics of epilepsy. My project was to define the genetic predisposition to seizures in patients who developed acquired seizures through birth trauma.
So we had a group of a large number of families, ascertained through Montreal Children’s Hospital and the Montreal Neurological Institute [7], who had hemiplegia due to birth trauma, and a good proportion of them had seizures as part of their disorder. So I was able to gather the families of these individuals and get electroencephalograms done on the parents to see if parents of children that had seizures were more likely to have abnormal EEGs than the parents of those who didn’t. This turned out to be true, and this laid the basis of looking at genetic predisposition to acquired seizures, which they carried on for many years. It was my first introduction to medical genetics research, really.
MM: That’s fascinating. I was wondering about this. You presented a paper, I believe, at a congress in Rome?
DR: Yes. When I was finished, I got my M.D. and master’s degree simultaneously and started an internship — at that time, everyone took a rotating internship at the Royal Victoria Hospital in Montreal, and I was able to get the paper based on this work accepted for presentation at the – at that time, it was the Second International Congress of Human Genetics in Rome [8], which was being held in September. So I started my internship a month early so I could take the month of September off and go to the meeting and travel around Europe. You can imagine with a group of interns who had already spent eleven months in the pit, they all disappeared when I arrived and I didn’t get much else done but take care of patients that year.
So that was a first entrance to it. And so I just pursued from then on — at that time, I wanted to become a medical geneticist. And the real in people who were involved in developing the field of medical genetics were internists: Victor McKusick [9], Arno Motulsky [10] , Alec Burn [11], Jim Neel [12], and others.
MM: I’m going to stop you right there. I want to go back just a little bit because we’re going just a little bit too fast. Let me just start with a couple of basic questions. Clearly, when you came to college, you already had an interest in science and a determination to go to medical school. Do you remember how that came about?
DR: Ever since I can remember, I wanted to be a doctor, for whatever reason. There was no one in my family who had ever been in the health sciences or health industry. In fact, I think I was the second person in our extended family to go to college. So it was something that was just there, for one reason or the other, and I was determined to get there.
MM: That must have been — and you came from a large family?
DR: No, I had two younger sisters, one six or seven years younger and another fifteen years younger. They ended up involved in nursing and occupational therapy, so we continued the health there. My oldest daughter is now getting her Ph.D. in public health at Johns Hopkins.
MM: Good for her. That’s great. Now, I know a little bit about the way medical genetics was developing at this time, and you’re going to have to correct me if I’m wrong. Obviously, there had been a lot of work done on mice and on fruit flies, some tracing of genes. Correct me if I’m wrong. The only way to discover whether or not there was a genetic history in humans was to do family tree studies. Isn’t that basically correct?
DR: Right. Pedigree studies [13] were the major way of determining whether a trait was inherited, and if you did a number of them, you could see whether it followed the typical Mendelian rules of inheritance. [14] And you could start going down beyond the phenotype to expand it by looking at things like EEGs or blood chemistry levels that were available, so that the pedigree became something that got expanded from just looking at physical features to laboratory features of an individual and getting closer to the gene as one went along in its development.
MM: Okay. Research was sort of the way you entered the field. You started to work with the mice. But you were much more interested in working with people.
DR: Right.
MM: Obviously, that would go along with your interest in medical genetics. Again, there wasn’t much available, other than genetic counseling, in terms of therapy or preventive strategies. You tell me. What could the field offer to patients at this time?
DR: Well, a lot of it was genetic counseling in terms of telling families what they had and how you can prevent having another child with it, in essence, what your risks were of having it. And Clark Fraser was one of the first people involved in setting up a genetics clinic as well, so he had a very active genetics clinic ongoing at Children’s Hospital in Montreal, and I spent my summers there and many evenings working on it. In fact, when I was still an undergraduate and a medical student, they would go off on meetings in the summer and leave me in charge of genetics in the hospital. So it was sort of exciting to be there, but it was something I’d been in for a long time.
MM: And clearly, you took to it really well.
DR: Mm-hmm.
MM: I did read your C.V. I noticed that in your second couple years of medical school, you seemed to have won prizes in virtually every field: pathology, surgery, ob/gyn, medicine, so you were clearly an outstanding student?
DR: Well, I got better as I went along. (he chuckles) During medical school, I really was able to get good grades and involvement. Now, it’s interesting that at McGill they gave out a lot of prizes for the best in every class, and I won them in neurophysiology and a variety of basic fields and then in the clinical specialties as well. Each year — it was funny. Each year, I remember coming in second in my class in general academic standing, but the person who was first was different each year, so when you averaged it out, I got the highest academic award in the graduating medical school. (he chuckles) So consistency and persistence, I think.
MM: (she laughs) That’s very cool. I like that. Okay. So you began a residency at Royal Victoria.
DR: Right, a residency at Royal Victoria, which included I think it was six weeks or two months at Montreal Children’s Hospital in pediatrics. So I was looking at both pediatrics and medicine at the same time, for very different reasons. One of the exciting times when I was an intern at Children’s Hospital – I think it was even when I was a senior medical student working there – Charles Scriver [15] came back from his fellowship with Charles Dent [16] in England and presented a whole new concept of cellular physiology that was just emerging there in the biochemical genetics and was something that really intrigued me and turned me on even further to a medical career in genetics. Charles tried to talk me into, at that time, doing something that was not invented yet and that was doing a joint pediatric and medicine residency, and it just seemed to slow things down. So I chose to do a medical residency, and as you’ll see later, became a pediatrician by adoption. (he chuckles).
ENDNOTES
[1] The history of the Department of Genetics at McGill University (located in Montreal) is linked with the appointment of Dr. F. Clarke Fraser in 1950. In addition to his research on the effect of environmental factors on birth defects, he brought together a core of geneticists to develop a research group in human genetics in the Department of Genetics and a division of medical genetics at the Montreal Children’s Hospital. In 1965, the Human Genetics Sector was created within the Department of Genetics. For more information on the history of genetics at McGill University, as well as key contributions made by the geneticists at McGill, please visit this website: http://www.mcgill.ca/humangenetics/history/.
An interview with Dr. F. Clarke Fraser is available in this collection.
[2] Teratogens are environmental factors which typically result in birth defects.
[3] A popular 1950s televised game show, What’s my Line? had a celebrity panel which questioned contestants in order to determine their occupation, or “line” of work.
[4] Dorothy Warburton is Professor of Clinical Genetics and Development in Pediatrics at Columbia University, where her work has focused on the epidemiology of chromosomal abnormalities and genetic factors associated with spontaneous abortion. She started one of the first clinical cytogenetics laboratories in the US in 1968. For a biographical sketch on Dr. Warburton, please visit http://www.columbia.edu/itc/hs/medical/bioethics/egir/cv/Warburton.pdf. For an example of her work, see Hassold T, Warburton D, Kline J, & Stein Z. (1984) The relationship of maternal age and trisomy among trisomic spontaneous abortions. American Journal of Human Genetics 36(6):1349=56, available at this URL: http://www.pubmedcentral.nih.gov/articlerender.fcgi?tool=pubmed&pubmedid=6517056.
[5] Leonard Pinsky, currently Emeritus Professor of Human Genetics at McGill, established a research program in human somatic cell genetics there and was named the first director of McGill’s Centre for Human Genetics in 1979.
[6] Julius Metrakos (1917-1998) was a human geneticist and one of the founding members of the program in human genetics at McGill University. Katherine Chrones Metrakos (1924-2005) also received her M.D. at McGill University. Using her expertise in encephalography and his expertise in human genetics, Kay and Julius Metrakos were pioneers in the genetics of epilepsy. For one of their important publications on the genetics of epilepsy, see Metrakos, K, & Metrakos, JD. (1961). Genetics of convulsive disorders. II. Genetic and electroencephalographic studies in centrencephalic epilepsy. Neurology 11: 474-83.
[7] For a brief history of the Montreal Children’s Hospital, founded in 1904, please see their website, http://www.thechildren.com/en/about/history.aspx. For more information on the Montreal Neurological Institute, founded in 1934, see: http://www.mni.mcgill.ca/about/accomplishments/.
[8] This meeting took place from September 7-12, 1961 in Rome, Italy. The first International Congress of Human Genetics met in Copenhagen in 1956, where the participants agreed to meet every five years after. The most recent Congress (eleventh) met in Brisbane, Australia in 2006. The Congress is now held as part of the umbrella organization the International Federation of Human Genetics Societies. For further information on the 1961 meeting in Rome, see Proceedings of the Second International Congress of Human Genetics: Rome, September 6-12, 1961. Rome: Istituto G. Mendel, 1963.
[9] An interview with Dr. Victor McKusick is available in this collection.
[10] An interview with Dr. Arno Motulsky is available in this collection.
[11] Alexander G. Bearn, now Professor Emeritus of Medicine at Cornell University Medical College in New York, is a human geneticist specializing in the genetics of metabolic disorders. He established the human genetics program at Cornell.
[12] James V. Neel (1915-2000), was the founder of medical genetics at the University of Michigan and the first scientist to recognize the genetic etiology of sickle cell anemia. For a brief biography of James Neel, please see Morton, NE. Recollections of James Neel. Mutation Research/Reviews in Mutation Research, 2003, 543 (2):97-104.
[13] The pedigree study is a technique developed by geneticists to investigate gene inheritance by researching family history. An introduction to pedigree analysis is available at Professor Phillip McClean’s website at North Dakota State University: http://www.ndsu.edu/instruct/mcclean/plsc431/mendel/mendel9.htm
[14] The laws of heredity elucidated by Gregor Mendel (1822-1884), an Augustinian monk, in 1865.
[15] An interview with Charles Scriver is available in this collection.
[16] Charles E. Dent (1911-76) was a British physician and biochemist who published many classic studies of the amino acids and linked hereditary conditions. For an example of his work related to human genetics, see Dent CE & Philpot GR. (Jan 23, 1954) Xanthinuria, an inborn error (or deviation) of metabolism. Lancet 266(6804):182-5. For a brief biographical memoir, please see Neuberger, A. (Nov 1978) Charles Enrique Dent. 25 August 1911-19 September 1976. Biographical Memoirs of Fellows of the Royal Society. 24: 14-31.
2. Arrival at Johns Hopkins; Developing Interest in the Genetics of Endocrine Disease; Early Work with Down’s Syndrome and Diabetes
MM: Interesting the way that happens. Can you explicate that a little bit more: biochemical genetics?
DR: At that time, the whole concept of how cell metabolism worked in terms of protein synthesis and the beginnings of DNA were there. At that same time, cytogenetics [17] had taken its turn and you realized that there were forty-six rather then forty-eight chromosomes. [18] And while I was still in medical school, at the end of it, Jerome Lejeune [19] found the trisomy in Down’s Syndrome. So it was just beginning to emerge; PKU therapy [20] was beginning to emerge; definition of these different biochemical bases. So it was just at the time I started clinical medicine training that all this was surfacing for the first time, in very different areas.
MM: Now, I have the impression – and this is just from listening to part of Dr. McKusick’s interview – that a lot of other fields were not quite sure what to do with genetics?
DR: That’s right. Genetics was sort of something that was very poorly developed in almost every place, except a few, in the country. I think that Victor was able to form a division at Johns Hopkins. At the same time, Jim Neel was forming a department at Michigan, and Arno Motulsky was forming a division at Seattle. And we had a division of medical genetics in Montreal with Clark Fraser. So it was just beginning to emerge. The American Society of Human Genetics [21] had just started in ’48. So when I got into this business, it was within the first five or ten years of the development of that field.
I remember going to present a paper at an early ASHG meeting which was held on the campus of the University of Colorado at Boulder. The entire meeting took place in one dorm, and I think there must have been about two hundred people there, which is quite a contrast to what it is now.
MM: Nowadays, you what have what? thousands, two thousand, three thousand?
DR: It’s probably about five thousand now.
MM: Really large meetings. Okay. So you were at Royal Victoria, but you were interested in going further.
DR: I was interested in getting my clinical training finished and then doing a fellowship in genetics. At that time, I wasn’t sure which direction to go in. Charles Scriver suggested I follow in his footsteps and go to work with Charles Dent in London, which sounded enticing to me. So on my way back from the meeting in Rome in ’61, I stopped by in London and interviewed with Charles Dent. And he had made arrangements a couple of years later for me to join him.
At that same time, I was in Montreal doing my residency, and I’ll always remember this. I was in the pediatric intensive care unit at Children’s Hospital, in essence, watching over the death of a child with cystic fibrosis. [22] I got a call, a page, and I picked up the call, and this voice said, “This is Victor McKusick in Baltimore.” I said, “Who’s kidding?” (he chuckles) He said, “No, it’s really me.” He said, “I heard about you from Clark Fraser. I’d like you to come down and work with me.” I said, “Well, I’m just an intern and committed to do a residency in medicine here the next year. Probably should finish it up.” And he said, “No, no. Come down and finish up your residency at Hopkins.”
To make a long story short, I stopped by and interviewed with the people running the medical residencies at Hopkins, which went very well. I was all set to move after my second year of internship residency at McGill to finish my third year at Hopkins and got a letter saying that there were no slots available and that no one had left as they had anticipated. This was in September, or something, so I quickly communicated with Professor Dent in London, and he didn’t have anything till the following year when he was expecting me.
So I was busily negotiating what I was going to do the next year when I got a telegram one day, I think it was November, saying that one of the senior residents at Hopkins had been drafted and could I take the place. (both laugh) So timing and chance is everything, so I ended up going down to Johns Hopkins to finish my last year of residency and got so excited by what was going on there and with Victor McKusick that I decided not to go to London and to pursue my interests at Hopkins. At that time, a new Ph.D. program had just been started in the School of Medicine in Human Genetics, and I was one of the first students to be able to take that and, in essence, to combine a fellowship and the Ph.D. in a three-year process.
MM: So you got your Ph.D. and you had your M.D. already.
DR: I had my M.D. and a masters and I got my Ph.D. at Hopkins.
MM: So you graduated from medical school in ’61.
DR: Correct.
MM: And the Ph.D., I think, was ’67.
DR: Sixty-seven. So I moved down to Baltimore in ’63 for my year of medical residency and then did three years of a Ph.D. fellowship program. When I first moved down to Baltimore, I stopped in Bar Harbor, Maine, where there was a very exciting Bar Harbor course, which I was one of the first ones there, maybe the third, or something. It was the time that the International Birth Defects Congress [24] was taking place in New York, so Victor was able to assemble all of the European people who were real pioneers in the field. So it was a very exciting two weeks for me.
MM: That must have been very heady. I can only imagine at this late date. You were able to meet who?
DR: Oh, Lejeune and de Grouchy [25] and all of the people — Dent and Cedric Carter and [26] many, many others, actually.
MM: What was the thrust at that point? Certainly, people were interested in deciphering the genetic code [27], right? That was still in progress?
DR: Well, it was still in progress. Of course, we were about ten years into the knowledge that DNA existed and what it was. It was a few years after the chromosomal revolution, if you will, so cytogenetics was a big topic at that time. And the issue of biochemical genetics and inborn errors in metabolism were very high. So those were the areas that were really beginning to surface at that time.
MM: It does sound exciting. Was there a perspective as to what this was going to — were people talking about genetic therapy at this point?
DR: Well, they really weren’t. That was more of a fantasy than anything else, because we really didn’t know what the genes were in terms of individual functions and what would be possible. But certainly, treatment of genetic disease was becoming very hot in terms of understanding the biochemistry of many of them and using dietary or pharmaceutical agents to, in essence, fool Mother Nature.
MM: Right, to manage the effects. So you came to Johns Hopkins. Can you tell me a little bit about working with McKusick, from your perspective?
DR: Victor was a very interesting person to work with, because his mind was going in fifty directions at once, and he was extremely busy. I found that the best way to deal there was to just create your own projects. And Victor was terrific in supporting you and finding the funding and getting you going. Or else, you became just sort of a valet in some ways of going through the other projects.
So I was really fortunate when I came to Baltimore. During my year of residency, by that time, I was very interested in endocrinology. I had been turned on to endocrinology by John Beck [28] and Martin Hoffman at McGill. It was a hot new field. It’s where the first real biochemical changes were being defined, the cytogenic changes. So I was very interested in the genetics of endocrine disease. I saw it as a way in which one could start understanding what led to genetic disease.
So I went around with the endocrinology people and did rounds with them during that time and, at the same time, went on rounds with the people in Dr. McKusick’s area in the Moore Clinic. [29] During my residency, I did two genetic projects, I remember. I came across a very unusual family while I was on my rotation at the V.A. Hospital in Baltimore, who had major clubbing of their fingers and changes in their face and was able to find this was a disease that had been described just before then, known as pachydermoperiostosis [30].
I was able to take this family and get everyone involved in the family. Went out and visited them, brought them in, and then brought them over to Johns Hopkins to what was then a beginning clinical research center and got a variety of things done on them, including cardiovascular studies, angiograms of their limbs, discovered what was causing the clubbing, and was able to put this together and had it published as the leading article in the New England Journal of Medicine while I was a resident. The interesting thing is that my mentor in health who was at the V.A. Hospital at that time and helped me through this was Victor McKusick’s wife, Anne McKusick.
The other topic I did was, I worked in the lab of Thad Prout [31], who was head of endocrinology at Hopkins at that time, trying to define what the trisomy in Down’s Syndrome did to gene dosage. And at that time, there was evidence —
MM: I’m sorry. Say that again?
DR: The effect of having an extra gene was, if you had three genes, would you produce more of a compound than if you had two. So we looked at some evidence that thyroid function was abnormal in Down’s Syndrome children. So I set up a screening area in the lab where we looked at some of the thyroid binding proteins to see – which had seemed to be in that area of chromosome 21 – to see if they were abnormal in Down’s Syndrome children.
It was not a project that led to any major information, but I remember this project very well because I went out to Rosewood Institute [32], which was an institute for mentally retarded, getting blood samples from a whole slew of Down’s Syndrome children. When I came back to Hopkins and was taking the blood samples up, somebody came running out and said, “John Kennedy has just been shot!” So the time when that was. That always kept that project well in my mind.
MM: And that was quite a tragedy, because Kennedy had a lot to do with funding for medical research.
DR: Right, and for mental retardation as well.
MM: Right, exactly. It sounds like you were quite busy as a resident. But you were interested in getting into endocrinology.
DR: I was interested in the genetics of endocrinology. It looked like a good model to study, and I was trying to combine my two major interests. One of the thoughts that I’d had, actually, when I was still a resident at McGill and working with the endocrinologist there, was trying to understand the genetics of endocrine disorders. One of my projects was to define the nature of diabetes. After reading the literature and what was not known about diabetes – at that time, diabetes was considered to be one disease, regardless of what the age of onset was or the complications. And after going through what little literature there was on this, I came up with a hypothesis that diabetes was a heterogeneous group of disorders and not just a single disorder [33], and that if we wanted to find a genetic cause, we’d have to look for multiple causes.
I put together a series of really evidence from the literature in terms of ethnic differences and diabetes syndromes due to different genes that caused diabetes and a variety of family and other studies. And when I got to Hopkins, I decided that this would be my Ph.D. thesis work at first. So I put together this paper and submitted it to an essay contest that the American Diabetes Association [34] was running at that time and won first prize for that paper [35].
I started working on that basis, and one of the things I thought that we could expand on was to document that the diabetes found in different ethnic groups, which seemed to be clinically different, was really different physiologically. So I developed a project where we were looking at the type of diabetes in the Amish [36], which Dr. McKusick was studying, and found a very homogeneous population with a very typical European form of diabetes and compared that to the Navajo Indian [37]. The Navajo Indian had a typical, in essence, Asian type of diabetes in which it was not very severe and really looked quite different. My brother-in-law at that time was a classmate of mine, who was doing a Public Health Service stint at the Navajo reservation in New Mexico, so we were able to go out there and devise a study.
MM: Was that Dr. Schimke?
DR: No, this was Dr. Saiki [38]. So we did this as a study, and it was able to show that, clearly, they were different populations with different types of diabetes. But I got sidetracked on the way, because while I was doing consults on the wards at Hopkins as a fellow, I came across this woman who was in hospital to have her mitral valve repaired. She was about four feet tall, and she looked just like a pituitary dwarf [39] with wrinkled skin and a high-pitched voice, except she was fully sexually mature and had a daughter who was normal. At that time, growth hormone deficiency was really not known, and pituitary dwarfism was only known to be a disease in which all pituitary hormones were deficient, so they were sexually immature, they had problems with their adrenals and their thyroid, et cetera.
But just that year, Berson and Yalow had defined the growth hormone immunoassay [40]. I looked at this woman and said, “Boy, she looks like she should have growth hormone deficiency, but she’s [sexually] mature.” I ran down the hall and got Tom Merimee, who had just set up the growth hormone immunoassay, and tested this woman, and sure enough she had isolated growth hormone deficiency. So we ran down to Victor’s office and Victor wrote a grant, and we got funded, and this is where I got into dwarfism research.
MM: Which certainly has been a major part of your career since. Okay. But we’re going too fast. I want to go back to diabetes for just a minute. Certainly, the genetic and family patterns of diabetes had been recognized before.
DR: Well, family patterns clearly had been recognized as far as that diabetes was a familial disease.
MM: It seems to me that merely from looking at clinical features of the disease, it would be understood that Asian forms, for example, were different from European forms.
DR: Juvenile onset diabetes was thought to be the same as adult onset diabetes at that time. They said it was multifactorial, that there were just a lot of different factors in it. And when you looked at the evidence that we had at that time — I think I was able to assemble about twenty different genetic syndromes that were being catalogued, which were clearly different genetic diseases, each of which had diabetes, or part of it, it became obvious to me there must be different genes that can cause diabetes.
Now, when I got up and started presenting these data at diabetes meetings and clinical research meetings, I was yelled down by diabetologists who were very upset that I was telling them they were not dealing with the single most common disease known to man. It took another ten years, really, before they officially recognized that Type 1 and Type 2 diabetes were different disorders. Now, of course, we’re finding many different genes causing each of these different forms of diabetes. We use this, I think, as a model to try and look at common diseases in man.
And it’s all coming back again, because there clearly is heterogeneity in most diseases, and glucose intolerance is simply a symptom rather than a disease. Anemia is a symptom rather than a disease. Yet we come back now to looking at some of the common diseases as being multifactorial and having multiple genes, in some instances additive — so it really is a combination of both things that’s happened in many of these common diseases, disease disorders.
MM: What we’re talking about — correct me if I’m wrong here. We’re talking about multiple genetic predispositions, essentially. Presumably, there may be a certain form of diabetes, perhaps juvenile onset, which is highly determined that at some point the body will fail to produce insulin, but this is sort of a process of the development because certain genes are mutated or not functioning in the way that they do in the normal human. Whereas –
DR: Well, as it turns out —
MM: I’m trying to make a differentiation here, that there would be other forms in which genetic patterns would fashion in such a way that the individual might or might not develop diabetes, depending on what their lifestyle was.
DR: Right. So there are various things — first of all, there are clearly single genes that produce diabetes, regardless of the lifestyle or regardless of anything else that happens. These are usually uncommon rare diseases associated with very specific molecular defects. So we have a group of different genes that can produce glucose intolerance, either because they decrease the production of insulin through a variety of mechanisms, or they affect the insulin receptor in some way at the cellular level. So there are those that are clearly single genes able to do it all by themselves.
So you can have that, or you can have a variety of minor things that will add together to interact with environmental factors that will produce diabetes. And there are clearly those in which environmental factors are the necessary thing to happen, be it obesity in Type 2 diabetes in some instances, perhaps a viral infection in some forms of Type 1 diabetes. In some instances, we now know, in Type 1 diabetes the genetic predisposition is due to the way one handles the immune system, and there are immune defects that lead to predisposition.
The twin studies [41] provided a tremendous amount of evidence for this. In fact, if you had Type 2 diabetes and you were an identical twin, your identical twin had almost a 100 percent chance of developing Type 2 diabetes. Whereas, if you had Type 1 diabetes and you were an identical twin, your identical twin had less than a 50 percent chance of developing diabetes. So clearly they were different disorders.
Now, as we learn the molecular basis of these, I think we’re able to understand more and more of how things can be additive and multifactorial on one hand, but there are totally different genetic defects doing it all by themselves on the other hand.
ENDNOTES
[17] Cytogenetics is the study of the structure of chromosomes. For more information, visit the website at http://www.pathology.washington.edu/galleries/Cytogallery/main.php of the University of Washington School of Medicine Department of Pathology.
[18] Joe Hin Tjio and Albert Levan demonstrated unequivocally in 1956 that humans possessed 46 chromosomes, correcting the previous work of Theophilus Painter in 1921, which had set the number at 48. See: Tijo JH & Levan A (1956) The chromosome number in man. Hereditas 42: 1-6. To read more about Tijo and Levan’s work, see Harper, PS. (2006) The discovery of the human chromosome number in Lund, 1955-1956. Human Genetics 119(1-2): 226-32, available at this link: http://www.springerlink.com/content/f7q425r58882nk33.
[19] Jerome Lejeune (1926-1994) was a cytogeneticist specializing in chromosomal abnormalities of genetic diseases. He identified the first chromosomal abnormality in humans, trisomy 21, which causes Down Syndrome, a genetic disease characterized by a range of cognitive and physical deficits. For more about Lejeune’s work, see: Knudson AG Jr. (1970) Jérôme Lejeune: The William Allan Memorial Award presented at the annual meeting of the American Society of Human Genetics, San Francisco, California, October 3, 1969. American Journal of Human Genetics 22(2):119-20.
[20] Phenylketonuria (PKU), an inherited deficiency of the enzyme responsible for metabolizing the amino acid phenylalanine, is characterized by neurological deficits and often mental retardation. PKU was one of the first diseases for which newborn genetic screening was mandated. For a history of PKU, please see Centerwall SA, Centerwall WR. (2000) The discovery of phenylketonuria: The story of a young couple, two retarded children, and a scientist. Pediatrics 105:89-103; available online at this URL: http://pediatrics.aappublications.org/cgi/content/full/105/1/89.
[21] For more information about the American Society of Human Genetics, please see their website at: http://genetics.faseb.org/genetics/ashg/ashgmenu.htm.
[22] CF is a chronic, progressive genetic disease disease of the mucus and sweat glands. It is an autosomal recessive disorder caused by mutations in the cystic fibrosis transmembrane conductance regulator (CFTR) gene.
[23] The Bar Harbor Course was designed as a intensive primer in medical genetics. It was the brainchild of Victor McKusick (see his interview in this collection) and John Fuller and led to a long collaboration between the Jackson Laboratory and Johns Hopkins University. For a history of the Bar Harbor Course, please see Guethlein LA. “The Bar Harbor Course”: A 30-year veteran in the teaching of human genetics. American Journal of Human Genetics 1990 January; 46(1): 192–207; available at this URL: http://www.pubmedcentral.nih.gov/articlerender.fcgi?tool=pubmed&pubmedid=2403754.
[24] This historic meeting,also called the Second International Conference on Congenital Malformations, was held July 14-19, 1963, in New York. See Papers and Discussions of the Second International Congress on Congenital Malformations. New York: International Medical Congress. 1964.
[25] For more information on Jean de Grouchy, please see Catherine Turleau (2004) In memoriam: Jean de Grouchy, 1926–2003. Annales de Génétique 47(4):435-437.
[26] Professor Cedric O. Carter (1917-1984) was director of the Medical Research Council’s Clinical Genetics Unit at the Institute of Child Health, London, from 1957 to 1982 and founder and first president of the Clinical Genetics Society in the UK (in 1970). For more information, see http://www.clingensoc.org/people/cedric_carter.htm.
[27] The genetic code is the series of three nucleotides (codons) that specify the sequence of amino acids for protein synthesis. The first codon was “deciphered” by Marshall Nirenberg (1927-_) at NIH in 1961.
[28] John C. Beck, a renowned endocrinologist, was appointed as the first head of the combined division of Endocrinology and Metabolism at McGill in 1957 and, in 1964, was named Physician-in-Chief at the Royal Victoria Hospital in Montreal.
[29] The Moore Clinic opened as part of the Johns Hopkins Medical University in 1915, and was later named after its first director, Joseph Earle Moore (1892-1957), a pioneer in venereal disease research and treatment. Victor McKusick became the director of Moore Clinic in 1957 and transformed it into one of the first clinics for genetic diseases. For a brief history of the Moore Clinic, please see McKusick, VA. (Nov 1974) Work and aims of the Joseph Earle Moore Clinic. Johns Hopkins Medical Journal 135(5): 291.
[30] Pachydermoperiostosis (PDP) is a rare hereditary disorder usually characterized by clubbing of the fingers, thickening of the skin of the face (pachyderma), and excessive sweating (hyperhidrosis).
[31] Thaddeus E. Prout (1923-_) is associate professor emeritus of medicine at Johns Hopkins.
[32] The Rosewood Center, established in 1888 to provide institutional care for the developmentally disabled, is located in a suburb of Baltimore, MD.
[33] For Rimoin’s pioneering work in this area, see Rimoin DL (1967) Genetics of diabetes mellitus. Diabetes 16: 346-351.
[34] Founded in 1940 to raise awareness of diabetes, the American Diabetes Association funds research into the disease and provides support for people with diabetes. For more information, visit their website at: http://www.diabetes.org/home.jsp.
[35] For the results of Rimoin’s research on genetics and ethnic differences in diabetes syndromes, see Rimoin DL. (1969) Ethnic variability in glucose tolerance and insulin secretion. Archives of Internal Medicine 124: 695-700.
[36] See: McKusick VA, Egeland JA, Hostetler JA, & Eldridge R. (1964) The distribution of certain genes in the old order Amish. Cold Spring Harbor Symposia in Quantitative Biology 29: 99-114; and Francomano CA, McKusick VA, & Biesecker LG. (2003) Medical genetic studies in the Amish: Historical perspective. American Journal of Medical Genetics. Part C, Seminars in Medical Genetics 121(1): 1-4, available online at this URL: http://www3.interscience.wiley.com/cgi-bin/abstract/104541009/ABSTRACT.
[37] See: Saiki JH & Rimoin DL (1968) Diabetes mellitus among the Navajo. I Clinical features. Archives of Internal Medicine 122: 1–5.
[38] John H. Saiki is Professor Emeritus of Internal Medicine at the University of New Mexico.
[39] Pituitary dwarfism is defined as decreased bodily growth with normal proportions, usually attributable to growth hormone deficiency.
[40] Rosalyn S. Yalow (1921-) and Solomon A. Berson (1918-1972) developed the radioimmunoassay of insulin and other peptide hormones, which they described in a series of classic articles between 1955 and 1960. Yalow received the Nobel Prize for this work in 1977. For their growth hormone assay, see Glick SM, Roth J, Yalow RS, & Berson SA. (Aug 24, 1963) Immunoassay of human growth hormone in plasma. Nature 199: 784-7. For a summary discussion of their work, see Berson SA and Yalow RS (Sept 21, 1967) Radioimmunoassays of peptide hormones in plasma. New England Journal of Medicine 277(12): 640-7.
[41] Twin studies, first suggested by Francis Galton (1822-1911), study the traits of identical and fraternal twins to determine the relative roles of genetics and environment in trait development. One of the best-known and most-respected, at the University of Minnesota, draws on a registry of 8,000 pairs of twins born in Minnesota from 1936 to 1955, plus some 1,200 pairs of male twins born 1971-81.
3. Studies in Growth Hormone Deficiency; Working with Isolated and Genetically Similar Populations; Washington University in St. Louis; Working in Africa
MM: Another question that occurred to me that I was hoping you would comment on is that, to some extent, genetics is dependent on finding these isolated populations or, I guess, genetically similar populations, for example, the Amish. I know there have been a lot of studies done in Finland, where apparently they have a high degree of genetic homogeneity [42].
DR: Right.
MM: And I just wondered if you could comment on the problems of studying genetics in these types of populations versus the usual American, which is getting more and more genetically heterogeneous all the time.
DR: Well, there are two ways of looking at the population work that I’ve noticed. One is that you’re dealing with a population that has undergone inbreeding, so that the chance of having homozygous recessive mutations popping up is much greater, and that’s what’s made the use of the Amish population and, say, the Finnish population so important, because these diseases came about because there were founders of the group who were small in number, their descendants intermarried over the years, and therefore, the chance of having one of the “bad genes” that everyone carries coming back together in a double dose is greatly increased.
So that was the real value of the Amish population to Victor’s early work on it was, here you had a population that was inbred, and you could trace the relatives back and reduce these recessive diseases that could easily be defined. And the same is true with the Finnish population. Leena Peltonen [43], for example, has made great strides using this population, and some of our Fellows who went back there have done the same. It’s really become very useful in molecular terms as well.
On the other hand, you can use these populations to study broader, less rare diseases because of the homogeneity of the population backgrounds. They share a common genetic background and quite distinct from others who have a very different common genetic background. You can see if sort of multifactorial traits are different, which is really what I try to do with the Amish studies as opposed to looking at single gene disorders.
MM: Thanks. That’s very clear. Okay. Well, I guess we should start talking about then the — dwarfism is the only term I have, so you’ll have to correct me if –
DR: That’s what we use.
MM: Okay. Which clearly became a major theme and interest in your career. What was it that attracted you? Was it similarity to the diabetes studies, the endocrine involvement?
DR: It was the endocrine involvement that really struck me first with discovering isolated growth hormone deficiency. And it was very exciting because two relatively young people at Hopkins, Tom Merimee and David Rabinowitz [44], were actively developing the growth hormone business from the endocrinological point of view, having the new radio immunoassay and the insulin assay, and I was able to work with them very closely to start going out and finding other people with these disorders. I would go around the country, Little People’s conventions [45], which I first looked at going to find these individuals. I remember once going up to New York with Victor McKusick for a meeting of some sort, and the Barnum & Bailey Circus [46] was in town at Madison Square Garden. We went down to the sideshow to try to find individuals who were, at that time, in what was really a freak show at that time, with various genetic diseases.
But we had started going to Little People’s meetings, and this is where we found some of these individuals. We were able to find a whole bunch of individuals who clinically fit the bill, brought them in, did studies on their endocrine metabolism and their growth hormone secretion and were able to define, as a group, that this was truly a disease, it was recessively inherited. And we went on to find that there were different forms of it from studying these patients. It was a time when we were developing new methods of studying growth hormone deficiency, that they developed with us. During this, we put together the arginine infusion study [47], which is still being used as a stimulus for growth hormone release as a diagnostic test. And the effects of glucose on insulin metabolism and of growth hormone and insulin working counteractive to each other.
At that time, Rabinowitz had just put forward the hypothesis we were dealing with, insulin being the hormone of feast and growth hormone the hormone of famine, and it really fed into what Jim Neel had proposed before about the thrifty genotype hypothesis [48] in diabetes. And it was all sort of coming together and really exciting to see this whole new field emerge.
During the time I was doing all this, as it became clear that we were dealing with these diseases, it occurred to me that the African pygmies [49] might be examples of growth hormone problems, because they looked just like — in pictures I had seen, at least, in National Geographic or wherever — they looked like proportionate dwarfs who are sexually mature. And Luca Cavalli-Sforza [50] was doing some of his population genetics work in the pygmies in Central Africa at that time, and Victor communicated with him when I brought him this idea, and I got a telegram back saying, “I’ll meet Rimoin in Bangi [51] in two weeks.” Well, I had no idea where Bangi was and what I’d need. I was in the midst of writing my final thesis, and I had no time to do anything. But we were able to get things together and figure out how you get blood samples back, because for the growth hormone and other protein immunoassays at that time, you had to have the samples frozen immediately or else they would deteriorate.
So in a matter of a short two weeks, I was able to find a visa to Bangi in the Central African Republic and figure out how we were going to take this stuff over. Well, first of all, we had to have IV bottles for infusions because you had to use insulin or arginine stimulation to test whether a pygmy, or anyone else, was secreting growth hormone. And at that time, all the IV bottles were in glass bottles, which were very heavy. Baxter [52] had just been testing the plastic sacks, so I got them to donate several cases of these sacks to take over.
How to get these samples frozen? They had just developed these ten-liter liquid nitrogen containers, which we now use to store frozen fiberglass, et cetera. At that time, they had just been developed to collect bull sperm in the field, and they were non-pressurized containers. So I was able to get a statement from the Federal Aviation Authority that it was safe to bring these things on planes. I got one or two of these containers. The liquid nitrogen would only last for a week or two, so I made arrangements with Air Liquide [53] in Paris to fill them up there. So we flew to Paris with these containers, went out to Air Liquide’s plant, and they filled up these containers. I was able to take them in the cabin of the Air Afrique plane, or whatever it was at that time, and we went down to Bangi and arrived there.
Luca Cavalli-Sforza and his Italian team met us at the airport, took us out to this jungle outpost that they had found, which was an old French museum outpost. And we set off going out to meet the pygmies. It was really fascinating because the pygmies, at that time, were considered subhuman by the Bantu [54], and they were, in essence, slaves. Yet they were the ones who were the true hunters of Africa. They’d go out and do the hunting and come back and sell the bad meat to the Bantu for spear tips and grain, et cetera, while they put on the charade of being slaves. But in essence, we had to go and learn how to barter, first of all, with the Bantu to be able to, in essence, rent their pygmies. That was in cash. Then with the pygmies, with materials, machetes, salt, cigarettes, eventually my mother’s old costume jewelry, so as to get them to cooperate. Informed consent issues that would exist today were not there. But they would all come back and line up to be part of the second test, so I figured they were happy with what they were getting. And there was no risk to these tests. At that time, we didn’t think there was a risk to those tests.
So in essence, the first time around, we would go out to these little pygmy villages, have to take a Jeep and cross the river on a mechanized raft, and get out there and set up these infusions, after we did all the bargaining. I could either hang these plastic sacks from the shutters on an old hut or have – I hired some of the Bantu to hold them for three hours. Or we tied them to a tree. We figured out some way. They had a portable generator that worked off of gasoline, so we could immediately centrifuge the samples and put them into the liquid nitrogen. So we had them frozen much faster than we did at the Clinical Studies Center at Hopkins, I think. But in those days, not knowing about HIV, which didn’t exist then, there was blood all over the place. It was really quite a scene.
But in essence, we got the studies done on a bunch of pygmies and went back to Baltimore, where Merimee and Rabinowitz examined the samples and found they made, totally, perfectly normal levels of growth hormone, yet their metabolic parameters were sort of like growth hormone deficients in terms of their glucose and insulin levels and fatty acid levels, et cetera. So we thought, Well, here we have a group of individuals who look like they have growth hormone resistance, perhaps.
At this time, I had to run back and finish writing up my thesis, which was called the “Genetics of Endocrine Disorders.” I started off with a catalog which I put together of all known genetic diseases of the endocrine glands, which eventually led to writing my first book with Neal Schimke on Genetic Disorders in the Endocrine Glands. [55]That had a section on all of the growth hormone studies and a section on the diabetes studies.
At that time, I was finishing my Ph.D. and looking at various jobs. I ended up taking one at Washington University in St. Louis [56], joining Bill Sly [57], who was a phage geneticist [58] who had just started a genetics program there.
MM: Let me just stop you for a second. So you’re leaving Hopkins now. Would you care to comment on what you think was — obviously, you were pioneering on your own in various lines of research. Was there anything that you learned in Hopkins that you took with you for the rest of your career? Any particular attitudes or skills?
DR: Yeah, I think that clearly one thing about Hopkins was that there was a feeling of excellence and pioneering, which was, I found, very exciting. And working with Victor McKusick, who just had such a fertile mind and was able to look at a problem and quickly figure out a way to approach it genetically and be persistent in his goals. Those are the things that I really took away from it. It was a tremendous influence on myself and my career as to how to approach a problem and the ability to be excited about transmitting this information and teaching it to other people. The fellowship program that he developed was exciting and it gave me something to emulate in terms of being able to pass this on to others.
MM: Now, at this point, genetics was still in a kind of a nascent stage, right?
DR: Right. It was beginning to become recognized as a clinical field, now more so in pediatrics, because of the inborn errors of metabolism in the chromosomal studies, which were then the hot areas of medical genetics. So this was in the late sixties, and there were people who were looking to develop genetics programs. I was looking to go back to Montreal. I looked at a variety of jobs, but this job at St. Louis, which was a top medical school with a great endocrine group there and an opportunity to develop the genetics clinic from scratch is what really appealed to me.
I must say I found the institution and the work and the people at the institution extremely exciting. I did not find the city and environment very exciting. (both laugh).
MM: I can certainly share those sentiments, unfortunately.
DR: I ended up there starting the genetics clinic. And at that time, we made two more trips to Africa. One trip was to find out, are the pygmies resistant to growth hormones? So we went back to Africa and went to the same villages and decided to give these pygmies a variety of tests, in terms of glucose and lipid metabolism primarily, and then give them three days of growth hormone injections; and then repeat the tests and see how they responded to the growth hormones, something we had experience doing in growth hormone deficients, as well as in normal individuals that the endocrine group had been studying.
I must say now that informed consent comes a little different in hindsight, because at that time, of course, they were using a pituitary derived growth hormone [59], and knowing the Creuzfeldt-Jakob issues that can come from that now – you know, twenty years later when they were discovered – makes you wonder what you had done. In fact, though I never got one there, both Rabinowitz and Merimee subjected themselves to the same studies as controls, and I guess we were very lucky that we didn’t have bad batches of the hormone.
But in essence, after that study, we found out that the pygmies were resistant to growth hormone [61] and all of its actions, and presumably, in its growth effect as well.
MM: Basically, nothing changed?
DR: Nothing changed. Their insulin levels didn’t change, their glucose didn’t change, their fatty acid levels didn’t change. It was really interesting to see that.
So we went back a third time to see, could this be environmental or was it genetic, and were able to talk to a group of the Bantu there and say, “Here you’re living in the same environment; we’d like you to do the same thing the pygmies did.” “Oh, we can’t do the same thing the pygmies did.” This was all done in my broken French. So we got them to participate in the study finally with negotiation and significant Central African francs. We got a group of Bantu to do the test of studying growth hormone secretion and resistance and found that they were perfectly normal. So it was not environmental, it was genetic.
That was really the end of my growth hormone studies, whereas these years I was evolving into the other aspects of dwarfism.
MM: That’s quite fascinating. You’ve sort of alluded to this a couple times. In a sense, the growth hormone and the insulin are kind of alternate forms?
DR: Right. First of all, insulin is the major hormone in glucose metabolism, so if you have glucose around, you produce insulin, insulin gets growth hormone and goes into the cell, and what it does is block fatty acids from coming out of the blocked fat breakdown. So you use glucose as your fuel. If you’re fasting, insulin goes down, you don’t have any fuel in glucose, so growth hormone comes out and growth hormone is a major lipolytic agent. So it breaks down fat in the cell and fatty acids come out to be used as the fuel, which is the basis of the Atkins diet [62].
And growth hormone, as it turns out, comes out physiologically in fasting and during sleep. So if you’re trying to diet – and this is what I’ve done personally in losing weight, is to get on an Atkins diet to make growth hormone come out and never exercise after you’ve eaten. Because if you exercise after you’ve eaten, you’ve got glucose to use your fuel rather than burning up your own lipid. It all started to make sense over the years, but it was these growth hormone deficients that led us on to the thought of how these things work, so it was really exciting.
MM: Well, it is quite fascinating. I mean, you can sort of see — you can imagine a kind of evolutionary development line for this as well.
DR: Right.
MM: Okay. So we’re on our way to St. Louis.
ENDNOTES
[42] The population of Finland, about 5 million people, is remarkably homogeneous, due to several centuries of isolation and intermarriage.
[43] Leena Peltonen-Palotie, a native Finn, is one of the pioneers in the use of genetically isolated populations in the genetics-based identification of disease genes. She is currently the MacDonald Distinguished Chair of Human Genetics at UCLA. For an example of her work, see: Ikonen E, Ignatius J, Norio R, Palo J, & Peltonen L. (May 1992) Huntington disease in Finland: a molecular and genealogical study. Human Genetics 89(3):275-80.
[44] See Rimoin DL, Merimee TJ, Rabinowitz D, and McKusick VA. (1968) Genetic aspects of clinical endocrinology. Recent Progress in Hormone Research 24: 365-437. Thomas J. Merimee is currently Professor of Endocrinology and Metabolism at the University of Florida.
[45] Meetings of the Little People of America, an organization founded in 1957 to improve the quality of life of people with dwarfism. For more information, see their website at: www.lpaonline.org.
[46] The Barnum and Bailey Circus, founded by the great showman Phineas T. Barnum (1810-1891) in 1870, was purchased by the Ringling Brothers in 1907. For more information, visit their website at: www.ringling.com.
[47] Use of the amino acid L-arginine to detect the level of growth hormone in the body. See Merimee TH, Burgess JA, & Rabinowitz D. Arginine infusion in maturity-onset diabetes mellitus. Defective output of insulin and of growth hormone. (June 11, 1966) Lancet 1 (7450): 1300-1.
[48] The classic paper in which James V. Neel discusses his thrifty genotype hypothesis, “Diabetes mellitus: A ‘thrifty’ genotype rendered detrimental by ‘progress’?” (American Journal of Human Genetics (December 1962) 14:353-62) is available at this URL: http://whqlibdoc.who.int/bulletin/1999/Vol77-No8/bulletin_1999_77%288%29_692-703.pdf
[49] This term refers specifically to various peoples of central Africa whose adults have an average height of 150 centimeters (4’11”) or less.
[50] Luca Luigi Cavalli-Sforza is an Italian-born population geneticist who has done extensive work on genetic variance in human populations and created the subfield of gene-culture coevolution. For his work with Rimoin, see Merimee TJ, Cavalli-Sforza LL, & Rimoin DL. (Feb 1972) Metabolic studies in the African pygmy. Journal of Clinical Investigation 51(2): 395-401, available online at this URL: http://www.pubmedcentral.nih.gov/articlerender.fcgi?tool=pubmed&pubmedid=5009122. An interview with Dr. Luca Cavalli-Sforza is available in this collection.
[51] Bangi, or Bangui, is the capital and largest city of the Central African Republic.
[52] Baxter Travenol, founded as the Baxter Intravenous Products Corporation in 1931 to provide supplies for hospital IV systems, is today a billion-dollar company with several subsidiaries, involved in the manufacturing and marketing of pharmaceuticals, hospital equipment, and medical supplies. The “Travenol” name was added in the 1960s.
[53] Air Liquide is an international company, founded in Paris in 1902, specializing in medical and industrial gases
[54] Bantu is a general term for more than 400 different ethnic groups in central and southern Africa, who are linked by a common family of languages. Both peoples and languages are quite diverse.
[55] Rimoin, David and Schimke, Neil. Genetic Disorders of the Endocrine Glands. St. Louis, Missouri: C.V. Mosby Co.: 1971.
[56] Founded in 1891, the Washington University School of Medicine in St. Louis is one of the leading medical schools and research centers in the US. More information can be found at this URL: http://medschool.wustl.edu.
[57] William S. Sly, MD, is Alice A. Doisy Professor and Chair of Biochemistry and Molecular Biology at Washington University in St. Louis.
[58] Phage geneticists study bacteriophages, the viruses that infect bacteria, to better understand genetics at a basic level.
[59] Starting in the 1960s, some 7,000 children in the US, and others in other countries, were given growth hormones derived from cadaver pituitary glands to treat conditions such as short stature and infertility. In the 1980s, however, it was discovered that a number of these patients had developed a fatal neurological disease (Creutzfeldt-Jakob disease).
[60] Creutzfeld-Jakob disease (CJD) is an extremely rare, degenerative, inevitably fatal, brain disorder that appears late in life, usually about age 60. However, in the 1980s, seven young adults were diagnosed with CJD. Research led to the conclusion that these young adults had been administered pituitary-derived grown hormones as children. More information about CJD is available at http://www.ninds.nih.gov/disorders/cjd/cjd.htm.
[61] Rimoin, David L. Hereditary forms of growth hormone deficiency and resistance. (1976) Birth Defect Original Article Series 12: 15-29.
[62] Dr. Robert Atkins (1930-2003) developed the Atkins Nutritional Approach, a weight-reduction diet consisting of a low carbohydrate intake in conjunction with a higher meat intake, in 1972.
4. Skeletal Dysplasia and the International Skeletal Dysplasia Registry; the Emerging Genetic Code and Genetic Counseling
DR: While I was still in Baltimore, one of the things that Victor had discovered in the Amish was a couple of forms of skeletal dysplasias: cartilage hair hypoplasia [63] and Ellis van Creveld Syndrome [64]. At that time, it was thought that, “well, there are only two major forms of genetic disproportion in dwarfism: achondroplasia and Morquio’s disease.”
MM: And could you define those?
DR: Achondroplasia is a form of dwarfism in which the most common disproportion form is a large head and short limbs. Morquio’s disease, which we now know is a very specific mucopolysaccharidosis [65], was characterized by a short trunk. Now, he [McKusick] discovered these two forms in the Amish; the Ellis van Creveld Syndrome, which had been described with polydactyly; and cartilage-hair hypoplasia, which was a new disease; and we were all, at the time, in the Moore Clinic, excited about these new developments and these diseases. And some new diseases started being described by the group in Paris, by Lamy and Maroteaux [66], who were describing some new variants of disproportionate dwarfism.
MM: Okay, and what we’re essentially talking about here is genetic failure of the skeleton to develop?
DR: Well, yeah, genetic mutations that will produce abnormalities in bone development and growth.
MM: Okay, so they also develop multiple digits, for example.
DR: It can do that depending on which of the genes, and we now know that there are several hundred different skeletal dysplasias. And these were all defined almost entirely on their skeletal radiology.
As we were going to Little People’s meetings to find proportionate dwarfs for the growth hormone studies, the majority of the people attending these conventions were disproportionate dwarfs, and still are. After awhile, they got upset that I was paying all the attention to the proportioned dwarfs and saying, “Why don’t you study us?” And I realized at that time that in these diseases that we were studying, no one knew anything. We didn’t even know the basic pathology of these conditions and what was going wrong in the bone. It seemed like just a very fertile area to get into to try and find out what the basic defects could be in these diseases.
When I went to St. Louis, I started focusing on this group of diseases, on the skeletal dysplasias, and started saying, “Well, we should be able to look, at least, at the pathology to see what’s going on in these bones.” What was written in the literature at that time in the pathology and orthopedic textbooks was totally wrong because the diseases were being misdiagnosed and didn’t realize they had heterogeneity. They were just learning at that time.
And I went to the pathologists at Wash U, who are excellent pathologists, and none of them were interested or knew anything about the growth plate, so I decided it was something I had to learn myself. And we started bringing in these patients with skeletal dysplasia from all over the place and got the collaboration of one orthopedic surgeon and then another one. And we did the brazen thing of bringing these people in, putting them under general anesthesia, and taking out a piece of their rib and their iliac crest to see if we can define their pathology and, in essence, were able, over the next number of years, to define the morphology [67] of the cartilage in each of these conditions, showing they’re really quite different. They had distinct characteristics, which might give us clues as to what the basic defect might be.
There was a woman by the name of Ruth Silberberg [68] who was one of the pioneering electron microscopists [69] at Wash U there, and I was able to recruit her to cut the sections and teach me how to interpret the ultrastructure of these conditions. So we did really many, many different forms, which we were able to get in and do these studies on.
MM: Okay, we’re talking about differences at the cellular level, really.
DR: The tissue and cellular level in the skeletal dysplasias, showing they clearly were different and they must have different pathogenetic and genetic mechanisms.
As part of this, I started putting this stuff away. We collected these samples of cartilage, and we carefully separated them out into frozen sections, which I put in liquid nitrogen and then in the Revco freezers to save for biochemical studies eventually – in formalin for the morphological studies; and in glutaraldehyde [70] for ultrastructural studies [71]; and of course, the radiology and clinical materials and some blood. This is how we started the International Skeletal Dysplasia Registry [72]. It just occurred to me to become a packrat and put this stuff away.
At the same time, I started fiddling in Bill Sly’s tissue culture lab and found that you could actually grow cells out of these cartilage samples, but they could never get them to stay as chondrocytes [73]. They all became looking like fibroblasts [74] after awhile. So during that time, that really didn’t seem to be much of a useful thing to do, although twenty-five years later, we found that it could be.
But in essence, what we did then was study purely the morphology of these conditions, because nothing was known about cartilage biochemistry at that time. But fortunately, I had put these things away, because twenty-five years later, we were able to pull them out of the freezer and use them for these studies as the technology advanced. It just became a very interesting part of the project to do, because very few people in the world were studying these conditions, and it was really trendsetting for us to do that.
MM: Okay, a couple of things. Possibly, one of the reasons that few people were studying them [the dysplasias] is because they were looked on as a kind of a disease of freaks, really, or a problem that was rare.
DR: Right.
MM: And applied to essentially – there’s a word I want to use — a marginal population.
DR: Yeah, they [the dysplasia cases] were a very few people; they were rare, and orthopedics would see them for their orthopedic problems, but they were not really concentrated in any one place, and very few people knew about them. There was a group internationally. Most of the work was being done in defining these diseases by Victor’s group and by the Maroteaux-Lamy group in Paris. Then there were a couple of other people, Wiedemann [75] in Germany, who had a young student, Jürgen Spranger [76], who came out, and some radiologists in Poland and in Germany.
But the exciting part, to me, was coming back after being away from Hopkins, the first year when I first got into this, with the First International Birth Defects meeting that the March of Dimes [77] had organized with Victor at Hopkins. And I have a picture of that year, which brings back many memories. But all of the people in the world who were involved in clinical genetics came to that meeting. It was an open, free flowing meeting of ideas and bantering. It really was an exciting time to be in that business. That was probably the most exciting meeting I’ve been to, to see a whole new field emerge.
And Victor had these things go on for the next five or six years in Baltimore. Then there was one that was held in Boston, and then I hosted the next one in Newport Beach. They were really exciting meetings in defining the clinical genetics of disorders. It was really the beginning of clinical genetics as a specialty.
MM: That’s fascinating, and we want to get into that more. Now, had he already started Mendelian Inheritance of Man [78]?
DR: Mendelian Inheritance of Man started off as a journal club.
MM: Ha ha! I love that!
DR: When I first came to Baltimore in ’63, one of the things I went to every month was the Medical Genetics Journal Club, which was held at Victor’s home. And people were assigned journals, so you’d get ten journals to cover over all the medical journals, and your job was to come in with a written summary of any article that had to do with genetics or genetic disease. And he accumulated these things and then published, I think it was in the early sixties, two volumes which were Medical Genetics ‘61 or ‘62, or whatever it was, in which all of these summaries were put together of all the articles that had been published that year in Medical Genetics. And then this led to combining these things into the first Mendelian Inheritance of Man.
MM: I knew it started out as sort of a — practically a mimeographed operation.
DR: It was really the first use of computers and desk printing technology in using these things.
MM: That’s great. By this time, though, by the late sixties, then, the code had been — Nirenberg [79] had come along with the [genetic] code.
DR: It was just beginning to emerge in terms of what the genetic code was and the issues of how proteins were coded for, and the concept of DNA and mRNA and RNA and protein synthesis was all becoming apparent at that time.
MM: So what were the goals of clinical genetics?
DR: Well, the goals of clinical genetics, I think, from the beginning were, one, define the disease, to be able to provide accurate genetic counseling. Just at that time, prenatal diagnosis started emerging through amniocentesis [80] in the late sixties, early seventies, mainly cytogenetically involved, but the thought of being able to do this for other diseases was clearly there. And then there were certainly a group of diseases that could be treated: inborn errors of metabolism, diseases that led to tumor formation in terms of prophylactic surgery, diseases of the heart, et cetera, et cetera. So there were many ways of treating genetic disease. So it was really — diagnosis, prevention, and treatment were the goals — and still are — in terms of genetic disease.
MM: But okay, we’ve got two scenarios here. One is genetic counseling, which happens prenatally in which parents will come in and have samples taken and amniocentesis done, and then you can determine whether or not the child may have certain chances of genetic disease.
DR: Right, for prenatal diagnosis. But even before that, genetic counseling was an active business in terms of saying, “Here’s what you have. Here are your risks of having children with this condition.” At that time, what they could do was decide whether or not to have children, or whether or not to marry each other, or whatever.
MM: But then in the early seventies, the possibility came that they could not have a child at all, that they could abort the child.
DR: Right. They could do that, and then the whole concept of carrier detection with Tay-Sachs [81] disease screening came to be, and at that time, I was able to, just as it started, recruit Mike Kabac [82] to join me in Los Angeles, where we started the California Tay–Sachs Disease Prevention Program [83].
MM: Okay, I didn’t know that. So the basic idea is to screen the susceptible individuals early in life?
DR: No, to screen them before reproduction if they’re at risk for having — carrying a recessive trait, like Tay-Sachs disease, so you can detect in the Jews – which was a high population of Tay-Sachs – detect those one in thirty who have the mutation, and if they married somebody who had the mutation, you knew they were a one-in-four risk. Then you could do prenatal diagnosis. So what Mike Kaback developed at that time was a whole new concept of how to prevent genetic disease, taking the newly emerging biochemistry knowledge and the emerging technical ability to do prenatal diagnosis.
MM: Well, okay, then the sort of second field here is, you have individuals who already have, perhaps, some sort of genetic problem. But normally, they’re not going to come to the clinic unless this has actually manifested itself clinically. Am I not correct about this?
DR: Right. Unless they have a family history of it.
MM: Well, even so.
DR: Well, it depends.
MM: I mean, I think now, in the 21st century, people are much more aware of it. They say, “Yes, I have a family history of breast cancer,” or whatever.
DR: Sure. Most of the genetic counseling at that time was for people who had an obvious genetic disease or had it in their close family, so they wanted to know if they were at risk for it. But then again, in many diseases, the other thing that a geneticist does, other than genetic counseling as a physician, is that you become the super specialist in the complications and natural history of that disease. So I became the general physician for people with dwarfism because I could then direct them to the specialist knowing what their predisposition to a variety of problems would be. And this is true for people who are biochemical geneticists dealing with PKU kids, or people dealing with cystic fibrosis or muscular dystrophy [84], or what have you.
MM: One thing that’s sort of becoming clear from what you’re saying is that developing medical genetics has broadened and deepened our understanding of pathologic processes to a degree that — I mean, I’ve been doing the history of medicine for a while and I haven’t thought about it in this fashion.
DR: It was a means of really trying to tie together the basic cause of the disease and its complications. The early mysteries there were, How can you have one gene defect cause so many different things going wrong in an individual? Look at sickle cell disease [85]. You have people who have anemia, they have strokes, they have problems in their spleen, they have problems in various parts of their body. That was the first disease known that we had a single molecular defect causing this. So you have the genotype and then the phenotype [86], and you have this big black box in the middle. That’s what we’re now deciphering is what lies in that black box. I guess that’s now called proteomics [87]. But it’s really being able to show through, first, pathological and then biochemical and pathophysiological mechanisms, how the gene produces the end result.
MM: Okay. So, you’re still in St. Louis. (she chuckles) You have an RCDA at this point as well.
DR: Yes, I got a Research Career Development Award [88] when I came to St. Louis and got my first NIH grant to do the studies on the skeletal dysplasias. That eventually evolved into a program project grant [89], and I’m happy to say that that’s just been renewed with continuous funding for years 31 through 35.
MM: Wow! That’s a really impressive longevity.
DR: So it’s been really the NICHHD [that] has been supporting us for over thirty years now.
ENDNOTES
[63] Cartilage hair hypoplasia is a hereditary form of short-limbed dwarfism due to skeletal dysplasia, first identified by McKusick and colleagues in the Amish in 1965.
[64] Ellis van Creveld syndrome (EVC), also known as ‘chondroectodermal dysplasia’, is a rare autosomal recessive genetic disorder characterized by short-limb dwarfism, polydactyly (additional fingers or toes), malformation of the bones of the wrist, cardiac malformation and other abnormalities. It was originally described in 1940 by Drs. Richard W. B. Ellis (1902-1966) of Edinburgh and Simon van Creveld (1895-1971) of Amsterdam.
[65] Mucopolysaccharidosis is an autosomal recessive disorder in which the lysosomal enzymes needed to break down sugar carbohydrates in the cell are absent or function abnormally.
[66] French physicians and geneticists Maurice Emile Joseph Lamy (1895-1975), and Pierre Maroteaux (1926-) identified a number of skeletal dysplasias and genetic disorders.
[67] Morphology is the study of the arrangement of plant/animal structure.
[68] Ruth Silberberg (1906-1997) was Professor of Pathology at Washington University in St. Louis. After becoming emerita in 1975, she emigrated to Israel, where she remained active as a researcher until her death. Her oral history is online at http://beckerexhibits.wustl.edu/mowihsp/words/OHSilberberg.
[69] The electron microscope, developed in the 1930s, uses electron beams to illuminate and magnify a scientific specimen. It has a much greater resolution than the light microscope and can magnify a specimen up to 2 million times; so it is invaluable in the study of cells, viruses, and other ultrastructural studies.
[70] A strong chemical disinfectant and preservative.
[71] Studies of biological structures too small to be seen with a light microscope.
[72] The International Skeletal Dysplasia Registry of patients with more than 380 types of skelelal dysplasia, conditions of abnormally developed bone and connective tissue, is now located at Cedars-Sinai Medical Center in Los Angeles.
[73] Chondrocytes are non-dividing cartilage cells.
[74] Fibroblasts are connective tissue cells that produce and secrete collagen proteins to synthesize and maintain the extracellular matrix.
[75] Hans Rudolf Wiedemann (1915-2006) was a pediatrician specializing in clinical genetics. After appointments at several German universities during the 1940s and 1950s, he became chair of pediatrics at Kiel University in 1961, from which position he retired in 1980. Wiedemann identified many genetic syndromes, some of which now bear his name. For a brief biography and bibliography of his work, see Opitz JM & Mullen P. (1992) Hans-Rudolf Wiedemann: An appreciation. American Journal of Medical Genetics 43(4): 737-739.
[76] Jürgen Spranger is Professor of Pediatrics at the University of Mainz and a senior member of the clinical faculty at the Greenwood Genetic Center in Greenwood, South Carolina. In 2004, he won the Otto Huebner Medal, the highest distinction awarded to German pediatricians, for his work on the genetics of skeletal dysplasia. Spranger and Wiedemann were the first to identify pondyloepiphyseal dysplasia congenita in 1966.
[77] President Franklin D. Roosevelt created the National Foundation for Infantile Paralysis in 1938 to help conquer polio by asking the public to send in dimes. Nicknamed the March of Dimes (later its official name), the Foundation played a pivotal role in the discovery of the Salk and Sabin polio vaccines in the 1960s. The Foundation then changed its focus to research on birth. More information about the foundation can be found at this URL: www.marchofdimes.com.
[78] Created by Dr. Victor McKusick, Mendelian Inheritance of Man is a comprehensive record of genetic disorders, each of which is given a identification number. Originally published as a mimeographed sheet in 1958, MIM developed into a published catalog based on computerized data in 1966, and appeared in successively more detailed editions before taking its current online digital form as OMIM in 1987. To learn more about MIM, see the Victor McKusick interview in this collection. Access to OMIM can be found at this URL: http://www.ncbi.nlm.nih.gov/sites/entrez?db=omim.
[79] Working in the Section of Metabolic Enzymes at NIH, Marshall Nirenberg (b. 1927) showed in 1961 how specific nucleotide sequences in DNA coded for specific proteins, thus deciphering the genetic “code”. He received the Nobel Prize for his achievement in 1968. For more information on Nirenberg, see http://history.nih.gov/exhibits/nirenberg/index.htm.
[80] Amniocentesis is a prenatal diagnostic procedure performed by inserting a hollow needle through the abdominal wall into the uterus and withdrawing a small amount of fluid from the sac surrounding the fetus; the fluid is then analyzed to detect chromosomal disorders.
[81] Tay-Sachs disease is an autosomal recessive genetic disorder in which harmful quantities of a fatty substance build up in tissue and nerve cells soon after birth, resulting in a rapid deterioration of mental and physical abilities. Most children born with Tay-Sachs die before the age of 5. Tay-Sachs is especially common among families of Eastern European and Ashkenazi Jewish descent, and was first described in the family of a rabbi in Brooklyn, New York. Dr. Warren Tay wrote the first report in 1881, and Dr. Bernard Sachs published a comprehensive account in 1887. More information may be found at this URL: http://www.ninds.nih.gov/disorders/taysachs/taysachs.htm.
[82] Dr. Michael M. Kaback is a professor of pediatrics, reproductive medicine, as well as the Chief of the Division of Medical Genetics at the University of California San Diego School of Medicine. He is also the director of the California Tay-Sachs Disease Prevention Program. See Kaback MM. (2001) Screening and prevention in Tay-Sachs disease: origins, update, and impact. Advances in Genetics 44: 253-65.
[83] The California Tay-Sachs Disease Prevention Program, launched in 1974 and based at UC San Diego, is a statewide effort designed to prevent the inherited genetic disorder of Tay-Sachs disease. The program provides community outreach services and testing and genetic counseling via statewide network centers in Los Angeles, Santa Barbara, San Francisco, Oakland, Sacramento, and San Diego.
[84] Muscular dystrophy refers to a group of hereditary muscular disorders in which the muscle fibers are unusually susceptible to damage and become progressively weaker.
[85] Sickle cell disease, or sickle cell anemia, is an autosomal recessive genetic disordercharacterized by the formation of an abnormal hemoglobin protein in the red blood cells, often forming them into the recognizable sickle (or crescent) shape. Patients suffer from anemia, jaundice, severe pain, and organ damage, due to abnormal blood flow and vascular blockage. Sickle cell disease is most commonly found in West and Central Africa and in African-Americans, but is also seen in the Mediterranean area, in the Near and Middle East, and in south Asia. More information can be found at this website: http://www.sicklecelldisease.org/index.phtml.
[86] The term genotype refers to the set of genes that an organism carries. Phenotype, on the other hand, refers to the observable manifestations of these genes – physical or behavioral. An organism’s genotype codes for specific proteins to produce the phenotype, but it is now understood that this is a complex process involving chromosomal interactions and environmental factors, as well as the genotype itself.
[87] Proteomics is a general term used to describe the study of proteins, their structure and functions. An organism’s proteome, the set of proteins it produces during its life, will differ from cell to cell and change continually through the proteins’ interactions with the cell’s DNA (genes) and with the environment.
[88] The RCDA (Research Career Development Award) (K series) is an NIH grant program designed to assist young scientists to begin their research careers.
[89] An NIH Program Project Grant (P series) supports an integrated, multi-project research endeavor, involving a number of independent investigators who share knowledge and common resources.
5. Leaving St. Louis; Establishing the Pediatric Genetic Division at UCLA
MM: Okay. That’s awesome. So, really, this has become your primary interest.
DR: That became my primary interest. At the same time, though, in the seventies, I was still very much involved in common disease genetics and, while in St. Louis, was looking at the diabetes studies and carrying them on at that time in the Navajo – the Amish studies had been pretty well done – and gathering the information using different genetic diseases to look at this.
Using that same model during the seventies when I came to Los Angeles, we had been publishing and getting more and more accepted the fact that diabetes was a heterogeneous group disorder. And Dr. Grossman [90] had just started a new peptic ulcer center at UCLA and asked me to consider looking at the genetics of peptic ulcer disease [91]. And I thought, Well, let’s approach it the same way. Let’s see if we can find heterogeneity in peptic ulcers, at least find some biochemical marker, like glucose intolerance or insulin levels. And got a grant to do that with him.
And at that time, the young medical student who wanted to come and take a fellowship in medical genetics, Jerry Rotter [92], was able to join me. I had money to hire him to do this project. And he became intensely interested in common disease genetics through this and really took over the field as I backed out of it. So we were doing that and found the various forms of heterogeneity there; and he’s now gone on to many other common diseases, such as coronary artery disease, hypertension, inflammatory bowel disease, et cetera, using that same model.
So that was in the seventies. I was really doing both, and at the same time, I was transitioning from an internist to a pediatrician. When I was in St. Louis, I saw that at Hopkins, the two genetics groups had evolved independently, Barton Childs’ [93] group in pediatrics and Victor’s in medicine. At that time, there was very little crossover, and I thought, If you’re going to start something new, let’s prevent that from happening. So I insisted on a joint appointment in pediatrics and medicine and really set the clinic up in pediatrics. And my first prime appointment was in medicine, and I was attending on both medical and pediatric services as a general physician in the teaching service. Then before I left St. Louis, my prime appointment switched to pediatrics.
And when I was offered the position and finally decided to come to Los Angeles to Harbor UCLA [Hospital] [94], I was given the option of which my prime appointment would be in, and I chose pediatrics because that’s where the action was at that time, and I really enjoy dealing with children much more than adults, and at the same time was able to attend on both services. That’s how the transition to that took place.
MM: Now, this does surprise me, because I’ve certainly worked in medical schools where disciplinary lines were rather strongly guarded and certainly people were happy to give joint appointments, but to allow you to cross the street in this fashion and make yourself a pediatrician –
DR: Well, no, I never considered myself a pediatrician and, I mean, dealing in the non-neonatal services, I found a lot of the issues in teaching were similar, but I never considered myself a real pediatrician.
MM: Okay. That makes it somewhat more clear.
DR: Now, there were others who did. Kurt Hirschhorn [95] took it much more seriously in terms of the training, and he started off in the same way, as an internist, and moved over to head of the pediatrics division. And he went out and got boarded in pediatrics. But it was really the genetics that was driving me rather than the pediatrics.
MM: I think that’s pretty clear. Do you want to comment anymore on your work in St. Louis?
DR: Well, St. Louis was a place where – it was an emerging medical school of great excellence. There were people there in endocrinology, such as Kipnis [96] and Daughaday [97], that really allowed me to build my endocrine knowledge. Philip Dodge [98] had come as the new chairman of pediatrics, and he was a neurologist, a well-known neurologist, from Harvard and allowed freedom to develop within his area; perhaps because he was not a pediatrician either, I was able to flourish in pediatrics there. I was able to really get my career started on the skeletal dysplasia work, and as I left St. Louis – I was only there for three years – I wrote an NIH grant which was funded. So I was able to get that accomplished.
I looked at a variety of jobs at that time. I think I looked at thirteen jobs in the three years I was in St. Louis. Then I chose to do the one at Harbor UCLA, which was a new emerging medical center; that was not the prime university spot, but there was a pioneering feeling there. We were dealing in these barracks in the back of this hospital, which was an old Port of Embarkation hospital in the Second World War. In essence, we were given a hammer and nails: “You can build your own labs.” Space was unlimited. And it was really an exciting place with young, bright people who were doing it. And Joe St. Geme [99] had put together, at that time, a cadre of us who were really an exciting group of young people, and it was a real freedom to do your thing, which really appealed to me.
MM: I knew they had this old hospital there. I didn’t realize there was still construction going on at that time.
DR: Well, they had all these barracks where the hospital was originally there. They had just put up the new hospital structure, the county structure, and gave the barracks to the research institute.
MM: Ha ha! I love it!
DR: So I had this barrack that I could design in any way I wanted to and built these labs.
MM: That’s wonderful! No wonder you came.
DR: I was looking at taking over Kurt Hirschhorn’s old job at NYU at that same Christmastime and came out to L.A. and saw the excitement in this new thing. Beautiful weather, walking on the beach. I got on a plane and went to New York in the midst of a snowstorm and saw dilapidated Bellevue [100] and some of the problems that existed, but in a very exciting environment there. And I took the sybaritic way out and came to L.A. And it was, really.
MM: I can see why you might want to do that. Was this then, the only – what else was happening in genetics in Los Angeles, or at UCLA, at that time?
DR: Well, at that time, there was not much happening in genetics at UCLA and in Los Angeles.
MM: I didn’t think so.
DR: There was a genetics developmental biology group at UCLA, which was highly regarded, but it was not well developed. Bob Sparkes [101] was there doing some cytogenetics. Barbara Crandall [102] was there doing some. She had just graduated. Stan Wright [103], who was the founder of genetics at UCLA, put together a group of people. As I remember, Bob was in medicine. And Steve Cederbaum [104] had just arrived in pediatrics to do biochemical genetics. But they were never brought together as a division. Stan died a year or so later, unfortunately, so there were really a number of good independent individuals at the main campus. At that time, the interaction between the campuses was far greater than it is now.
Sherm Mellinkoff [105] did a great job of bringing together people from all the campuses and integrating them. And I was able to start a new division at Harbor from scratch. I brought a fellow with me, Dave Hollister [106], from St. Louis at that time and then a year later was fortunate to recruit Mike Kaback to join me. And he came and started the California Tay-Sachs Program, which he really did and developed that as the model for preventing genetic disease throughout the world.
And we, together, built a division. We trained a lot of people. I brought Larry Shapiro [107] to come and join us. He was a first year medical student at UCLA when I was a first year faculty member there teaching a course in medical genetics and helped turn him on to the field. We became good friends. He went on to NIH with Liz Neufeld [108] while I started this division at Harbor. He was set to go back to St. Louis as a faculty member, but we enticed him to come to Harbor UCLA instead.
And we built up a fantastic division there with a large number of faculty. Started a training program with Mike back in the beginning of the seventies. We had the only training program, really, in medical genetics at UCLA. And I got a grant for that and trained a lot of people from around the world and America, which was really an exciting time. And built up to a large faculty. I’m proud to say that, I think, three of us from that division won the Mead Johnson Award [109] in pediatrics. There were five of us, [who won] the Ross Award from the Western Society for Pediatric Research [110]. It was really an exciting time. And we had congenial relationships with the people at UCLA, mainly in running the medical school course together, which we started. But it was when I decided to come to Cedars-Sinai that I was able to integrate the UCLA teaching programs.
MM: Okay. Let’s put that on hold for a while. Tell me a little bit about your family. You’re married to another physician, or to a teacher?
DR: Well, I’m in my second marriage right now. I was married to a woman who was working at McGill at the time I was a medical student. We were married and actually stopped — my first visit to Johns Hopkins for interviews was on our honeymoon. Then we lived — we moved to St. Louis and had our daughter Annie and then came to L.A. in 1970 when she was about six weeks old. And then about eight years later, got divorced.
I was very fortunate after that time to marry Ann Garber, who was, at that time, a genetic counselor. After we were married, she got her Ph.D. in public health at UCLA as a reproductive geneticist. Then we had two children together, Michael, who is now a freshman at Middlebury College [111], and Lauren, who is a junior in high school. And Annie, as I mentioned, is now getting her Ph.D. at Hopkins in public health.
MM: Continuing the family tradition.
DR: Well, she got turned on to medicine and sciences, not until she was past her college career and went to the Peace Corps [112]. I think having been turned on to some of the African things from my experience, and when she was in the Peace Corps got interested in public health.
MM: Okay.
ENDNOTES
[90] Morton I. Grossman (1919-1984) was Professor of Medicine at UCLA and the Wadsworth Veterans Administration Hospital, and founder of the UCLA Center for Ulcer Research and Education.
[91] Peptic ulcer disease (PUD) occurs when ulcers, or mucosal erosions form in the stomach lining or in the small intestine. Because these areas are highly acidic, the ulcers are usually very painful and discomforting. Some 80% of peptic ulcers are linked to Helicobacter pylori, a spiral-shaped bacteria commonly found in the stomach.
[92] Jerome I. Rotter is now Director of Research and Co-Director of the Medical Genetics Institute, Director of the Division of Medical Genetics (Department of Medicine) and Director of the Common Diseases Genetics Program at Cedars-Sinai Medical Center. He holds the Board of Governors Endowed Chair in Medical Genetics at Cedars-Sinai.
[93] An interview with Barton Childs is available in this collection.
[94] Harbor General Hospital was opened by the City of Los Angeles in 1946, which had bought the Port of Embarkation Hospital built three years earlier to house WWII wounded soldiers evacuated from the Pacific. A formal operating agreement with UCLA was signed in 1958.
[95] An interview with Kurt Hirschhorn is available in this collection.
[96] David Kipnis is Distinguished Professor of Medicine, Molecular Biology, and Pharmacology at Washington University School of Medicine, where he served as chair of the Department of Medicine from 1972 to 1992. He has been nationally recognized for his pioneering research into diabetes, insulin regulation, and the metabolic effects of hormones and was elected to the National Academy of Sciences in 1981.
[97] William Daughaday is currently Professor of Medicine at the University of California Irvine School of Medicine. As a fellow in endocrinology at Washington University School of Medicine in 1949, he conducted research on human growth hormone with Nobel laureates Carl and Gerty Cori.
[98] Philip R. Dodge, MD, was Chair of Pediatrics at Washington University in St. Louis from 1967 until 1986. He is currently Professor Emeritus of Pediatrics and Neurology.
[99] Joseph W. St. Geme, Jr. (1931-86) was chair of the Department of Pediatrics at UCLA from 1977 until 1985 and a national leader in pediatric research.
[100] Located in New York City and first opened in 1736, Bellevue Hospital Center is America’s oldest public hospital. Affiliated with New York University School of Medicine since 1968, Bellevue remains a major research center.
[101] Robert S. Sparkes, MD, is Professor Emeritus of Medical Genetics and Pediatric Genetics at UCLA.
[102] Barbara F. Crandall is Professor Emerita of Pediatrics-Genetics at UCLA.
[103] Stanley W. Wright, Professor of Pediatrics at UCLA, was one of the pioneers of medical genetics at that institution. He was involved in the assessment of the effects of atomic bomb radiation on pregnancy loss in women at Hiroshima and Nagasaki and in early studies of mental retardation due to PKU and other genetic disorders. See Wright SW & Tarjan G (April 1957). Phenylketonuria. AMA Journal of Diseases in Childhood 93(4): 405-19.
[104] Stephen D. Cederbaum is Professor in Residence of Psychiatry and Biobehavioral Sciences, Pediatrics, and Medical Genetics at UCLA. His particular research interest is in deficiencies of the enzyme arginase, a critical component of the urinary system, and in the other enzymes of arginine metabolism.
[105] Sherman M. Mellinkoff, MD, (b. 1920) was the dean of the UCLA School of Medicine from 1962-1986, a period of major growth for the institution. He is currently Professor Emeritus of Medicine and pursues research interests in the digestive diseases.
[106] David W. Hollister (1941-91) was professor of medicine, pathology and microbiology, and pediatrics at the University of Nebraska Medical Center, where he founded the connective tissue disease laboratory, at the time of his death.
[107] Larry J. Shapiro, MD (b. 1946) became internationally known in genetics and, after serving as Chair of Pediatrics at UCSF for 12 years, was appointed Olin Distinguished Professor of Medicine and Dean of the Washington University School of Medicine in St. Louis in 2003.
[108] Elizabeth F. Neufeld (b. 1928) is Professor and former Chair of Biological Chemistry at UCLA.. She received the National Medal of Science in 1994 for her work on the mucopolysaccharide disorders, a group of lysosome storage disorders which includes Hurler syndrome and Hunter syndrome. For a short biography with citations to her classic papers, see: Kresge N, Simoni RD, and Hill RL. (May 2007) Lysosomal storage diseases: The work of Elizabeth F. Neufeld. Journal of Biological Chemistry 282 (20): 15-17.
[109] The E. Mead Johnson Award for Research in Pediatrics was established by the Society for Pediatric Research in 1939. Considered the most prestigious award in pediatrics, it honors both clinical and laboratory research achievements in the field.
[110] The Ross Young Investigator Research Award is presented to young faculty members who have completed exceptional research in a pediatrics-related field.
[111] Middlebury College is a liberal arts college located in Middlebury, Vermont.
[112] The United States Peace Corps, established by President John F. Kennedy in 1961, recruits young Americans to live and work in developing countries. Volunteers work in the fields of education, youth outreach, community development; business development; agriculture and environment; health and HIV/AIDS; and information technology. For more information, see: www.peacecorps.gov.
6. Developments at Cedars-Sinai, Los Angeles; Emery and Rimoin’s Principles and Practice of Medical Genetics
MM: Okay, we’re continuing. This is the second part of our interview with Dr. Rimoin, and today is the 6th of February. It’s about ten minutes to two. Good afternoon, Dr. Rimoin, again.
DR: Good afternoon.
MM: Let’s see. I’m going to push that a little closer so I can see you a little better. I’m going to pick up the camera because, first, I want to show you the beautiful view from the window. For those of you who are viewing the tape, we’re only on the fourth floor of Cedars-Sinai, but we do have — a little smoggy today, but a nice view of the hills behind, the Hollywood Hills, I guess. Here are a very interesting pair of shoe braces, and there’s a story about those which you told me last time. Do you want to tell me that one again?
DR: Approximately twenty-five years ago, a Mexican family, a well-to-do, educated family, came to see me when I was at Harbor UCLA Medical Center down in Torrance with their two-and-a-half-year-old daughter who had achondroplasia. She was wearing these heavy braces that an orthopod in Mexico had put on to prevent bowing. They were really concerned because this child had not yet walked, and in looking at these braces and seeing how heavy they were, I suggested that they take them off and leave them with me and go back to the hotel. I predicted that the child would walk that night, and sure enough, she did. So I keep those braces as a remembrance of this.
Approximately fifteen years later, this family showed up on my doorstep to say, “You told us something might be done for achondroplasia. Has anything happened yet?” And I had just come back from Europe, where I attended the first Congress on Achondroplasia [113] and heard all the leg lengthening talks and visited Professor Villarrubias in Barcelona [114], who was ready to start his procedure. So Lucia turned out to be our first patient to have extended limb lengthening. She is now in her late twenties, gainfully employed, out dancing every night, and enjoying life.
MM: Wow, that’s really a great story! I love it. Okay, that does bring us up to limb lengthening, but let’s catch up on where we were last time. You came here to Harbor General in 1970 from St. Louis.
DR: Yes.
MM: And we talked about that last time. It was a fairly productive and exciting time. Now, there was a period of time when you didn’t have the appointment at Harbor before you came to Cedars-Sinai?
DR: No. I was at Harbor straight through. I don’t know if we talked about building a division of genetics there under Mike Kaback and Larry Shapiro and Jerry Rotter.
MM: We talked about that.
DR: Okay, I left Harbor to come to Cedars as Chair of Pediatrics and build this Genetics Center in 1986. And at the same time, Mike Kaback went down to San Diego to become Chair of Pediatrics there, and Larry stayed on with the division for another few years before he went up to UCSF to become chair.
MM: Okay, okay, now at some point — I’m picking up from our discussion of last time. You referred to — you had taken a set of frozen samples and sort of stowed them away, and you said they became interesting about twenty-five years after you did it.
DR: No, actually, at my time in St. Louis and then shortly after arriving in California, we started the International Skeletal Dysplasia Registry and started collecting samples on various dwarf children that we had encountered, both specimens that were sent in and those that we went in electively to obtain. We continued collecting these over the years, and when it became time to have useful technology to do biochemical and molecular studies, we were able to go back in the freezer and retrieve these samples and do very productive work on defining the molecular defects on those samples. So some of the ones that we are using today to define molecular defects were actually collected and frozen away thirty years ago. So it pays to be a packrat.
MM: I guess so. And going through your research papers, I find mention of a number of syndromes that you’ve identified over the years. A lot of this is from going back and looking at samples. Is that right?
DR: Well, most of the syndromes are looking at patients and finding new diseases on the basis of unique characteristics in patients or their families, some are defining the biochemical basis. But most of them by clinical description, and sometimes corroborating it by differences in their morphology on samples.
MM: How useful are animal models in this then?
DR: Well, the animal models have been quite useful in defining disease. I think they’re going to become even more useful in the future. For years, we concentrated on trying to find homologies between natural mouse mutants and the human disease. Very few of them, actually, showed up and were clearly comparable in terms of their genetics and morphology. The problem is, the bones do look different in mouse and man.
MM: I would imagine.
DR: Both from the radiographic and the morphologic point of view. But there are similarities. In the last couple of years, using knockin and knockout mice [115] and creating mutations in known genes, we have been able to study a variety of different models. In fact, people define the basic defect in the human disease based on the mouse model. And I think that, now we know that total human genome and mouse genome and going on to look for synteny [116] between them, I think the mouse models are going to become even more important, and hopefully, even more so in defining the pathogenesis of these conditions.
MM: Okay, now, a lot of lay people have been sort of conditioned by learning about the human genome and hearing about the work in identifying all these genes and the DNA sequences, to the idea that there is one gene for one disorder. I’m sure this is not true. In fact, basically, your whole career has been about showing that, in fact, a particular type of common disorder can be linked to, can actually have a variety of etiologies, beginning with genetics and then also, frequently, with environmental interaction playing a role as well.
MM: So, identifying the human genome, where exactly do we go from there? Do we start looking in the genome, mapping the genome, do we start looking there for genes that we already know about? What exactly do we do now?
DR: Well, we can do two things. One, if we identify where a gene is that we know about and understand the pathogenesis, how that gene operates, we can maneuver through that mechanism to try and find out how to interfere with the actions of that gene. On the other hand, we can search for new genes that were not known, and we’re doing that through a variety of techniques. We’re looking in libraries of tissues in which genes specific to that tissue have been identified and seeing if we can find diseases that fit that, or really, through mapping studies. And what we’re finding is that many common diseases, as well as rare diseases, are due to many different genes.
Sometimes, they are totally different genes in a heterogeneous state, and sometimes in the more common diseases, we’re finding there are complex interactions between different genes that will lead to mutation, to the disease. This is going to be very important in terms of, not only prevention of the disease, but in finding therapeutic agents, because each particular distinct gene mutation will require a different form of therapy. This is where the whole area of pharmacogenomics [117] will come in, in that one size doesn’t fit all and that one will have to define the very particular type of disease, the diabetes type 23B that you’re treating, which might well have a different cause than 22A.
MM: So it’s really a much more rich picture, a more complex picture that was envisioned even ten years ago.
DR: Right. Much more so. And I think what we’re going to be finding is that when we look at drug reactions, that when we say 60 percent of people with the disease will respond to this agent, perhaps it’s 60 percent of the people respond 100 percent of the time and 40 percent respond none of the time because they have a different disease.
MM: Looking over your career here in Los Angeles, what do you think is the most interesting thing that you’ve done or that you’ve worked on?
DR: I think the whole issue of the skeletal dysplasias, to me, over this thirty-year period, has been fascinating, because I started off looking at purely clinical features and then was able to dig a little deeper with the pathological features, then finding the appropriate collaborators as biochemistry and molecular technology improve. And really taking it from the patient to the gene, and, hopefully, going back again, has been fascinating to me and shows you that if you stick to it and have some persistence, eventually it might pay off.
MM: Okay. Now, the book that the camera is sitting on is a classic work, Emery and Rimoin’s Principles and Practice of Medical Genetics [118]. I believe the fourth edition came out just a couple of years ago. Is that right?
DR: Just a month ago.
MM: Just a month ago! Okay, I’m a little behind. And it’s now in three volumes.
DR: Three volumes.
MM: Right, the fourth edition. The first book was in two volumes. Did I get that right?
DR: The first book was in two volumes, as shown right here. It stayed in two volumes through the first three editions, and we’re now up to three volumes.
MM: Okay, I’m just focusing the camera. I’m missing one of the volumes because I have it right here so I could put the camera on top of it. But we have here, then, the four editions of Emery and Rimoin. Could you tell me just a little bit about how you came to write this book and why you were thinking it was important to put the principles and practice of medical genetics down on paper?
DR: Well, Alan Emery [119] is a geneticist who was a year or so ahead of me in the Ph.D. program under Victor McKusick at Johns Hopkins. We got to know each other then. And he went back to Manchester and then to Edinburgh to become the Chair of Human Genetics. And during the fifteen years after that, we saw medical genetics grow into a real clinical specialty, although it had not yet been clearly recognized as such, and felt there was a need to compile all this new information that was coming about.
Alan had been contacted by Churchill Livingstone Company [120] in Edinburgh and asked to meet with me at the International Congress of Genetics [121] that was being held in Moscow that year, which we were attending. So in 1978, Alan and I met in a little coffee room in the side of a floor in the Hotel Rossija in Moscow and conceived of this book and thought of how we might put it together. I think it was the following year we went to Edinburgh to finalize the details.
With the emergence of genetics as such a huge field and so broad, we’ve eventually been able to add several other editors onto the list to cover certain areas, such as Mike Connor [122] in Glasgow [123] and Reed Pyeritz [124] and Bruce Korf [125] here in this country.
MM: And it’s meant to be a comprehensive reference work?
DR: It’s supposed to be a comprehensive reference work covering all of medical genetics, from basic parts to looking at it in a system by system fashion, similar to the other principles and practices that have been defined over the years.
ENDNOTES
[113] The First International Achondroplasia Conference took place in Rome, Italy in 1986.
[114] Jose Maria Vilarrubias is Professor of Orthopedic Surgery at the Dexeus Institute in Barcelona, Spain. See: Vilarrubias JM, Cavaliere P, & Ginebreda I. (1988) Lengthening of the lower limbs and correction of lumbar hyperlordosis in achondroplasia. Basic Life Sciences 48:357-71.
[115] Mice developed in the laboratory by either deleting [“knockout”] specific genes in specific chromosomal locations, or by inserting [“knockin”] substitute genes into the chromosome. The mice are useful models for the study of genetic inheritance and variance, and for gene therapy experiments.
[116] Synteny describes the relationship between two loci (site of a gene on a chromosome) on a single chromosomal pair.
[117] Pharmocogenomics is the study of genetic variation in drug response. Researchers attempt to correlate the efficacy or toxicity of specific drugs with single-nucleotide polymorphisms (SNPs) or differences in gene expression, usually in lab animals. Pharmacogenomics is a branch of pharmacology.
[118] Emery, Alan E.H., and Rimoin, David L., Principles and Practice of Medical Genetics. Edinburgh and New York: Churchill Livingstone, 1983 (4th edition 2002).
[119] Alan Eglin Heathcote Emery (b. 1928) is Emeritus Professor of Human Genetics at the University of Edinburgh and has current affiliations with Duke University and with the European Neuromuscular Centre.
[120] Churchill Livingstone is an imprint of Elsevier Ltd, global publishers of health and medical books, journals, and CD-ROMs, including reference works and textbooks. The original Churchill imprint dates back to the medical publisher John Churchill, who began his firm in 1728.
[121] This was the 14th International Congress of Genetics in 1978. The first International Congress of Genetics met in London in 1899; subsequent Congresses met at irregular intervals until after WWII. At the 8th International Congress in Stockholm in 1948, it was agreed to meet every five years, the schedule that has been followed to the present. The 20th International Congress of Genetics will meet in Berlin in August, 2002. For the proceedings, see: Vartanian ME, ed. Well-being of Mankind and Genetics : Proceedings of the XIV International Congress of Genetics. [August 21-30, 1978, Moscow] Moscow: MIR Publishers, 1980.
[122] J. Michael Connor is Professor and Head of the Department of Medical Genetics at the University of Glasgow and the co-author (with Malcolm Ferguson-Smith), of Essential Medical Genetics (5th edition 1997).
[123] The University of Glasgow, founded in 1451, is the fourth oldest university in the English-speaking world.
[124] Reed E. Pyeritz, MD, is Professor of Medicine and Genetics and Chief of the Division of Medical Genetics at the University of Pennsylvania School of Medicine. His principal research interests include Marfan syndrome, an autosomal dominant genetic disorder characterized by disproportionately long limbs and cardiovascular abnormalities, including the fatal form of the syndrome in which the aorta slowly enlarges and dissects.
[125] Bruce Korf, MD, PhD, was appointed Finley Professor of Medical Genetics and Chair of the Department of Genetics at the University of Alabama Birmingham in 2003. He is a nationally known expert on neurofibromatosis, the group of autosomal dominant genetic disorders in which tumors develop in multiple areas on the body, including on the skin, on the bones, and along many of the nerves.
7. The American Board and the American College of Medical Genetics; Further Developments at Cedars-Sinai and UCLA; The GenRISK program
MM: Let’s talk a little bit about some of the other sort of field-essential work that you’ve been involved with. At about that same time, were you not working with the creation of the American Board of Medical Genetics?
DR: Yes. For the same reasons that we felt a textbook was necessary to cover these areas, we felt that there needed to be some recognition of medical geneticists as a specialty in medicine and have some way to certify that individuals were qualified to practice medical genetics. I was asked by the American Society of Human Genetics at that time to serve on, or to put together a task force to look at medical genetics and its certification. I think Arno Motulsky was the president at that time.
And we had a task force that recommended the formation of a Board, and I was then asked to put the Board together. So we got an extremely influential and active medical advisory board to get together. We met over several years to see if we could put together a specialty Board in Human Genetics and Medical Genetics.
At that time, the American College of Medical Specialties [126] was not allowing any new specialties to join, so we had to look for other means of forming a Board and felt it would be most legitimate to form this independent Board if we used an excellent well respected examination company to actually produce the exam. So we contracted with the National Board of Medical Examiners [127] to do that. And fortunately, we received a grant from the Kaiser Family Foundation [128] that allowed us to finance the beginning of this, and since then, the Board has been self financed. So we created the first Board exam in, I think it was 1980, and certified a large number of people. Then the Board [members themselves] took the next exam and had to find a whole new set of people to serve on the examination Board.
MM: That’s sometimes difficult in the beginning of a new field?
DR: Well, there were enough people around. We certified enough that we could then go and see if they would certify us.
MM: I see.
DR: And the Board has grown into a totally independent entity. Then, in the early 1990s, through efforts of a number of individuals who were running the Board by that time, we finally gained acceptance into the American Board of Medical Specialties. And then I was able to take it to the Council of Medical Specialties Societies [129] to really firmly establish medical genetics as a full specialty Board.
One of the controversial issues in this was the question of how genetic counselors were to be treated, because when we formed the first American Board of Medical Genetics, we wanted to be all-inclusive and had examinations for medical geneticists, Ph.D. geneticists, genetics counselors, and then cytogeneticists and biochemical geneticists. So everyone would take a general exam. Then each of the subspecialists would take their own exam.
When we reached agreement with the American Board of Medical Specialties to join, they said, “We only certify M.D.s.” And I pointed out to them that, in fact, Ph.D.s were being certified by the American Board of Radiology as radiation physicists, so they agreed that we could maintain certification for the Ph.D.s, but not for the lab specialties and not for the master’s counselors. And the master’s counselors were very upset in terms of, Were they going to be excluded? They felt it was extremely important to have the Board and the College clearly support the genetic counselors in forming their own organization. And as a result of this, we also created what is now known as COMGO, or the Council of Medical Genetics Organizations[130], in which genetic counselors, as well as nurses and physicians and Ph.D.s, will each have a word in the formation of new ideas in medical genetics.
I think this has been excellent for both groups, actually. I think the genetic counselors have achieved a little more autonomy through this. And that clearly, medical genetics is now well recognized as a medical specialty.
MM: And certainly, not unknown in the formation of professional fields where there’s a certain amount of conflict between the physicians and — for example, nurse anesthetists and anesthesiology are one example this brings to mind. People who are master’s prepared or technically prepared and who perform a lot of the crucial functions but don’t have medical degrees and haven’t invested themselves in a medical career. I take it was this tension not present?
DR: Oh, I’m sure there was tension between them saying, “If you wanted to be part of the America Board of Medical Specialties, then you should get an M.D.” But clearly, there was no feeling, I don’t think, among the physicians that they wanted to let the genetic counselors go flying off on their own. It was simply a matter of, this is the only way we could achieve certification as a medical specialty, which had major import in terms of reimbursement, for example.
MM: Sure.
DR: Serving on CPT [131] committees, et cetera. That’s where the college came in.
MM: Yes, I want to talk about the college because — I hope you’ll be able to explain that to the uninformed person. There’s an American Society, an American College — the American Board is a certifying and examination entity. That part I think we can understand. But exactly what role do these two organizations play, the American Society and the American College?
DR: The American Society of Human Genetics was formed, I think, in 1948, at which time the people who were doing research in human genetics got together and formed a Society to promote the field of medical genetics — really, human genetics then. Medical genetics was not even a term being used at that time. And it became the sole official genetics organization in the country. By the time we reached the late seventies and the medical aspects of genetics were building up and which led to the formation of the Board, the Society had formed the clinical committees and things to deal with various clinical issues; however, their main focus has always been research.
So about a decade later, it became obvious that the Society could not really concentrate on being a medical specialty society as well as a research society and would, in essence, need to break off a clinical entity just as existed in other medical specialties, such as the Society for Pediatric Research [132] as opposed to the Academy of Pediatrics [133] and so forth.
And I was asked at that time to take on the next role of forming the American College of Medical Genetics as a medical specialty society, and we were able to do that. One of the real hot items at that time was the feeling that the physicians were not paying attention to reimbursement and the problems of the clinical lab directors. And the clinical lab directors were about ready to go off and form their own organization. I felt this would really be splintering too much, so one of the first things I did was to insist that the formative board of the College be made up of clinicians of all specialties, including pediatrics, medicine, and OB, and representatives from the clinical laboratory specialties, such as biochemical, molecular, and cytogenetics. So we set up a board, and then when we created the bylaws for the College, made sure that there was equal representation and firm representation from each of these groups.
This, to me, was very exciting, because we were able to really bring together all of these disparate groups and prevent them from splintering, which I think would have really wrecked the cause. And Mike Watson [134], who was one of the leaders of the clinical lab specialty groups at that time, came into the group and saw that we were really working to create an equal citizenship and society, and he has become the most active person in the College and now has become its first executive director. So I’m feeling very good about maintaining that unity between the lab and the clinic people.
MM: Okay. That’s excellent. You came to Cedars-Sinai [135] in 1986?
DR: Correct.
MM: And do you want to talk a little bit about why you decided to make this change?
DR: Well, actually, I looked to make a move in about 1983 when the chair at Cedars-Sinai became available, the chair in pediatrics, that is. Cedars-Sinai was one of the other UCLA medical centers, which we had significant contact with, especially in promotion committees, and such. It was formed as a community hospital and would become an academic center. And there was really nothing much going on in genetics here as well. And I felt that times were changing in what was originally a county hospital situation at that time with no limitation on space and the dollars flowing freely, things started tightening up and looked to see a new environment where we could really make things happen. I thought we had reached our limit at Harbor and would start going downhill.
So I actually was chosen for this position and negotiated it in 1983. And just as we were about to sign a contract, the California MediCal [136] laws changed, and they created a tsar of MediCal, or Medicaid as it’s known throughout the country, and Cedars did not get a contract. For pediatrics, that would have been unquestionably disastrous, so we parted company at that time and I stayed on at Harbor.
Two years later, my daughter was born at Cedars-Sinai and I spent a good deal of time in the hospital for a few days and saw how much I liked it and how much my original thoughts of what could happen here were true and approached the CEO of the hospital at that time. They were about ready to fill the position, and they stopped the search and instated me in the position. So I was able to just really retrieve what we had negotiated, which was really to build a genetics center, as well as take over the Department of Pediatrics.
MM: And what had happened with MediCal?
DR: MediCal — Cedars eventually got a contract. They got some HMOs involved and the patient population returned. So my job was to build the Department of Pediatrics, which was a department with just a few full-time people, into a full academic specialty — we have about fifty people on full-time faculty with all the specialties covered — and to develop the genetics center, which was well funded with startup funds. And we were able to develop it as a cross institutional department with positions in pediatrics, medicine, obstetrics, et cetera. And that really worked.
It was to be a center, in essence, without walls. We developed lab space. We were able to bring over a number of people from Harbor. Jerry Rotter, who was our Fellow back in the seventies, had stayed on and became my co director and really has taken on day-to-day management of the center. And we set off to recruit a large number of individuals, many of whom are still here fifteen years later. It’s been a lot of fun in bringing this together to see the genetics program become so broad, both in clinical and in basic research and in service provision, and to see the pediatric department grow alongside it. This is something that I think has been extremely valuable to me to see how one can take a private hospital and make it become a center for learning in genetics.
At that same time, I had had a training grant from NIH, which we had achieved at Harbor back in the early seventies. And when I came up, Dr. Elizabeth Neufeld had just arrived as Chair of Biochemistry at UCLA, and we put together the thought of creating an integrated, intercampus training program in medical genetics. And I brought the training grant into the show; she had some money from a foundation. We brought in the clinical geneticists of UCLA Westwood and formed this new intercampus medical genetics training program, which is now in about its fifteenth year. And it’s been highly successful and has really served as the nidus for bringing young people and faculty together from all aspects of the genetics communities of the three major UCLA hospitals.
MM: So it allows them to pursue both research and clinical training?
DR: They can do research and clinical training. We also recognized that clinical genetics was becoming a specialty in itself and there were people who were going to be geneticists without doing research. So we created a three track system, which was fairly unique at the time, in which people could choose to do research and clinical work. They could choose to do clinical work only and go for their Boards. Or they could choose to do a pure research postdoctoral fellowship.
MM: So, a lot of choices were available to them.
DR: Yeah.
MM: And they could work both here and at UCLA.
DR: Well, they could choose their primary mentor at either UCLA, Cedars, or Harbor. We started a weekly teaching program that meets at UCLA and has two or three hours of lectures, followed by the genetics seminar, and brings in people from all over the three campuses, as well as now students from the Cal State Northridge genetics counseling program [137], and others. And there are a lot of people on the campus who are basic scientists, who want to get experience in medical genetics as well.
MM: Sure. Yeah. It’s clearly the coming field. Now, I’ve come across a couple other things that you seemed to have been involved in originating at Cedars-Sinai. The GenRISK program [138]?
DR: The GenRISK program is a program that Jerry Rotter and I thought of many, many years ago as common disease genetics became more prominent. And that is, What do you tell a person who has a family history of coronary artery disease, breast cancer, ovarian cancer, what have you, as to what their risks are of developing this disease? Many of these people think their risk is 100 percent if they’ve had several relatives with it, when in fact, it’s certainly less than 50 percent in most cases.
So we conceived of this program, and one of our graduate fellows a few years ago, Maren Scheuner [139], started the real clinical arm of this program, in which we take families, people from off the street, in essence, who have a family history of disease, get their family history, look for new markers, molecular or biochemical, that can help distinguish which of the types of disease they have, and whether or not they’ve inherited the mutant gene, and then teach them ways for early detection or change of lifestyle, drugs, etc., to prevent that from happening. I think this should serve as a prototype for many, many different diseases.
MM: Yeah, it sounds like it. Are people sort of self referred?
DR: Yeah, many of them are self referred. Many of them are referred by their physician or internist, what have you. One of the major problems that still exists with this type of program is the question of insurance risk discrimination. So if something comes in and you finally have a marker for breast cancer, are they going to be denied insurance, or [have it] made much more expensive.
The Kennedy-Kassenbaum Act [140] several years ago tried to avert this from happening, but all it did was say they couldn’t deny you insurance but they could charge you whatever they wanted for it. Plus, it doesn’t affect half the people in each state. So each state has been going on doing this. Now there are several bills in Congress that should lead to avoidance of risk discrimination in insurance and in the workplace, and this will allow this program to really flourish. Now some people want to come in and pay for it in cash so it won’t go on their medical records.
MM: Would it — how do I say this? Surely it must be true that there are a number of people going around in business out in the world who are at risk for various genetic illnesses and have no idea that this is so.
DR: There isn’t anyone in the world who doesn’t have a risk for a genetic condition.
MM: That’s what I would think.
DR: It’s a matter of which one you have.
MM: So do you envision this becoming eventually part of a routine health screening program?
DR: Oh, I think so. And I think that as the results of the genome project unravel and cheap technology unravels, there will be multiplex screening for multiple diseases, then counseling and risk aversion because of that.
ENDNOTES
[126] Dr. Rimoin is probably referring here to the American Board of Medical Specialties, a non-profit organization established in 1933 to oversee the standards set by the 24 medical specialty boards for the evaluation and certification of physicians.
[127] The National Board of Medical Examiners, founded in 1915, is a non-profit organization which develops and administers exams to medical students to assist state medical boards in assessment of new applicants. It also administers post-licensure exams to physicians.
[128] The Henry J. Kaiser Family Foundation carries out and sponsors health policy analyses and research and assists to disseminate public health and health policy information to physicians and to the public. Originally established by Henry Kaiser in 1948, it was substantially reorganized and its goals redefined under CEO Drew Altman in the early 1990s.
[129] The Council of Medical Specialty Societies (CMSS) is an organization of major medical specialty groups, organized in 1965, to represent the views of specialist physicians on health policy, medical education, and accreditation.
[130] The Council of Medical Genetics Organizations, consisting of 17 professional groups, represents the views of genetic physicians, scientists, and genetic counselors, on research, academic, certification and clinical practice policies. It was established in 1992 as described by Dr. Rimoin.
[131] CPT codes were developed by the American Medical Association, starting in 1966, to standardize codes for medical services and procedures, allowing for more accurate and reliable communication between physicians, other health professionals, insurers, and patients. The CPT codeset is edited and maintained by a 17-member panel of physicians and health professionals appointed by various organizations. The codeset was accepted as the national standard for electronic health care transactions by the Department of Health and Human Services in 2000.
[132] The Society for Pediatric Research, founded in 1929, works to encourage young investigators to work on research problems that will improve children’s health.
[133] The American Academy of Pediatrics, founded in 1930, is the professional organization of American pediatric professionals; in additional to representing their interests, it disseminates information on child health, helps to set standards for medical education in pediatrics, and promotes research into pediatric health problems.
[134] Michael S. Watson, PhD, was Director of Clinical and Molecular Cytogenetics at Washington University in St. Louis from 1986 until 2000, when he became Executive Director of the American College of Medical Genetics. He retains an Adjunct Professor appointment in Pediatrics at Washington and also serves currently as Director of the National Coordinating Center for Regional Genetics and Newborn Screening Collaboratives.
[135] Cedars-Sinai is the largest nonprofit medical center in the western United States. Located in Beverly Hills, California, it was founded in 1902. For more information, visit this URL: www.csmc.edu.
[136] The California Medical Assistance Program, or Medi-Cal, is the state of California’s Medicaid program and provides health care services to eligible low-income individuals and families.
[137] The Genetic Counseling Program at California State University Northridge is an interdepartmental two-year Masters of Science degree program cosponsored by the Department of Biology, the Department of Educational Psychology and Counseling, and the Department of Special Education. In 2008, it was forced to stop accepting new students due to statewide budget cuts.
[138] The GenRISK Adult Genetics Program, established within the Division of Medical Genetics at Cedars-Sinai Medical Center in 1986, specializes in the identification of genetic risk factors for common adult-onset diseases.
[139] Maren Scheuner, MD, MPH, was Director of GenRISK from 1995 to 2003. Currently (2008), she is a Senior Research Scientist at the UCLA Center for Health Policy Research, where she is working, under the CDC Family History Public Health Initiative, to develop and evaluate a practical and valid “genomics tool” that uses family history to assess disease risk and inform prevention strategies.
[140] Also called the “HIPAA” (Health Insurance Portability & Accountability Act of 1996), the Kennedy-Kassebaum Act, co-sponsored by Senators Edward Kennedy and Nancy Kassebaum, requires: 1) Improved efficiency in healthcare delivery by standardizing electronic data interchange and 2) Protection of confidentiality and security of health data through setting and enforcing standards.
8. GenoMed; Treating Skeletal Dysplasias; Future Possibilities
MM: Right, we’re starting another tape and it’s February sixth again, still. And recording okay, good. Also since you’ve been here, you were involved in GenoMed [141], which I guess is still ongoing. It’s changed its name?
DR: GenoMed is not ongoing anymore.
MM: Then I really want to hear about it because it’s true history.
DR: Well, GenoMed was a company that we formed as a means of trying to do a commercial approach to defining the genes for common diseases and using them as markers. And Jerry Rotter and Jake Lusis [142] at UCLA and Nathan Fischel [143] and I here, so it became part of this and Cedars took an equity part in it and we raised some venture capital to start this to see if we could get more funding to do this by that route.
Well, to make a long story short, the venture capitalists came in, took control of the company, brought in a CEO from northern California to find that they wanted to have the company up there. Moved the company to the north after several years, increased the earnings ratio to the point that they ran out of money, and it’s now Abbott Lab’s [144] major genome facility. So this was a very interesting education in the corporate world and what not to do next time.
MM: It certainly sounds like it. Was the goal to identify new genes?
DR: To identify genes, often using mouse models to do that in terms of being able to define the various forms of risk factors, just as we’re doing in our common disease research and as we’re eventuating into the GenRISK program. It’s really just another means to raise money to do the same research.
MM: Okay, let’s go back to skeletal dysplasias. Clearly, they fascinate you; you’ve spent so much of your time [on them]. Are all these essentially forms of limb malformation?
DR: Well, some of them are limb malformation, some are trunk malformation, some are both. There are several hundred different forms of the diseases. And I guess they fascinated me because no one else was bothering with them. It seemed to be something that I could make a unique contribution to, and there are so few people in the world dealing with them, and rather than get into a field that everyone was dealing with because it was more common. So I guess you can look at both aspects of this in defining what you do.
But they really served as a real model for me to be able to bring together research potential, find new things, with taking care of patients who really needed help. These individuals had no one they could turn to who knew anything about them. Most physicians would sort of look at them and shrug. And knowing so many of them and treating so many of them over the years, I became really familiar with what their complications were. And many times, the most grateful patients are those that you can’t do anything for, but to reassure them you know what you’re doing. And that’s really been true in so many of these disorders. And now that we’re reaching the point of really doing something and finding these disease markers, it’s really important.
To me, one of the important things that happened to me this year was being named an Honorary Life Member of the Little People of America. And I just received a plaque from them, and it really brought tears to my eyes to see how these folks really appreciated what we had done. I consider many of them good friends. I know that in developing the limb lengthening program, I angered many of them who are opposed to invading their society and trying to sort of eliminate it in many ways, but I stuck to my guns and felt that if somebody wanted it, they should have availability of it. If they didn’t like it, they shouldn’t be forced to do it, but they shouldn’t be able to force their views on anyone else. I guess that’s the way I look at life in general. And to get over this while still pursuing this career and then realizing that, yes, it does have a place in certain people’s lives made me feel very good, I’d say.
MM: From what I’ve read about it, the people who have had limb lengthening have responded very positively. It’s really changed their lives.
DR: Yeah, in many of them. We’re just completing a questionnaire study of our patients to see that. Next week, I’m going to the Hastings Center in New York [145] on a conference called Surgically Shaping Children to see a discussion on should parents be able to, in essence, force cosmetic surgery on their children? Limb lengthening [146] in dwarfs is one of the topics which we’ll be addressing. And it’s going to be very interesting to see the ethical back and forth of this.
MM: Yes, this is very interesting. It has, obviously, lots of implications and in different areas. We ought to explain a little bit more clearly what limb lengthening is about.
DR: Okay, well, limb lengthening – there are various techniques of limb lengthening. It was originally defined in the early fifties, really, in modern technology by an orthopedic surgeon in Siberia, whose name was Llizarov. And he developed a technique, usually based for people who had asymmetry of their limbs, either because of an injury or through some congenital malformation. What he was able to do was, in essence, develop what he called “bloodless surgery,” in which he did small percutaneous burr holes [147], broke the bone subperiostially [148], and then put in a device where he had pins above and below the break, and telescoping rods in circles; and in essence, was able to stretch the fracture one millimeter a day over months and gradually could get up to fifteen centimeters of increased growth.
So this was the beginning of these techniques. Then he expanded it to do bilateral limb lengthening in dwarfs. Various other people took this on. And what’s involved is using a variety of different devices, that is, telescoping rods that will allow you to stretch the fracture line.
The person whose technique I thought made most sense was a man by the name of Jose Maria Villarrubias in Barcelona, who was using an offshoot of this technique, but he did several different things. One was, he changed the angles of bones so the center of gravity of these people changed from the front to back as well as up and down, and he was able to change their balance quite a bit. He was able to cut ligaments and tendons and get rid of the swayback [149] they had, which is one of the factors leading to the paraplegia [150] they might develop later on in life. So there were a lot of corrective issues involved in this technique.
When I went to visit him, the most important thing to me was all his patients were smiling, whether before or after the technique. And I sent some orthopedic surgeons over to Barcelona to learn the technique, brought him over here. We did our first patients. We do very few of them. I think we’ve done less than fifteen over fifteen years and turn away far more people than we take. It’s very labor-intensive. But every single one of them has said, “I would definitely do this again if I had the chance.”
MM: So what happened was that you have to break the bone and attach these rods and pins, and then, essentially, new bone tissue forms around the break.
DR: Right. It forms in the fracture space. You just spread the fracture space. When you reach the limit – the limit usually is based on the soft tissues – you can’t stretch the nerves or vessels or muscles too much. And then you stop and put opposite pressure on so the bone fracture fills in, forms new bone, and then you get them back. And it really is amazing to see this happen.
MM: It is quite astonishing. It’s quite time consuming.
DR: It’s very time consuming. It takes two to three years by the time you’ve done the femurs, the tibias, and the upper arms. But it really does make a major difference in these people’s lives. And the technique that we use causes very little pain, for the most part. The complication rate is 100 percent. There’s always a complication, and multiple ones. And you never end up at exactly the place you thought you were going to be at the time, so you have to be very committed to do this.
Many places who do this type of stuff do it in a vacuum, just an orthopedic center doing it without people understanding the problems of dwarfism, without doing psychological testing, et cetera, which I think is wrong. And many of them will start in childhood, and I think it’s so consuming that we won’t start until they’re twelve or fourteen years old, when the child can make the decision rather than the parents.
MM: Yeah, I think that does sound more reasonable. Do insurance companies cover this?
DR: Well, we’ve been able to get several insurance companies to cover it because of correcting the deformity and correcting the risk for spinal stenosis.
MM: So the Little People of America, now this is an organization that has been around for some time.
DR: Yes.
MM: I don’t recollect – I think the forties.
DR: Yeah, I think the mid-fifties is about when they formed.
MM: And of course, like with many such organizations, a part of the organization is being proud – is to enhance pride in their identity.
DR: Absolutely.
MM: And to essentially establish themselves as viable, as people with a difference, but the difference is like different eye color, or something like that.
DR: Right.
MM: So they do see the surgery as a problem.
DR: Well, certain ones do. When you say an organization doesn’t like it, it’s certain individuals that are often the vocal ones that make it sound like the organization. But is this any different than using growth hormone in growth hormone deficient dwarfs? This is the same situation that exists in the deaf community with cochlear transplants [151]. So I think that what the basis of all of this is, I think, is one can state one’s values and one’s purposes as long as something is safe and medically proven or under close supervision. Then each individual has to make their own choice.
MM: That makes sense. Are there other therapies which are available to the people with the dysplasias, with the different kinds of dysplasia at this time?
DR: Well, we can treat their various complications. We can treat the bow legs, the back problems, we can treat their eye problems. There are a variety of different other tissue components that are involved in these conditions specific to each particular disease. Treating their complications or preventing them has been something we’re mostly involved in. Whether it will come to a point in time when we can correct the molecular defect or the biochemical defect that results from a molecular defect — I think it will come. I think that achondroplasia will be treated medically rather than surgically in the next ten or fifteen years.
MM: They found the specific gene that’s responsible for much achondroplasia, right? It’s just in the last decade.
DR: Well, achondroplasia is one of the many types of skeletal dysplasia, and they found a gene that was responsible for achondroplasia in about 98 to 99 percent of the cases, it’s actually the same mutation. And now our group and others are doing research in seeing, How can you get around this sort of super turned-on receptor? which is what happens.
MM: Super turned-on receptor. That’s really interesting.
DR: Yeah, the receptor is turned on to its max rather than turned off, which often happens. That probably generates a gene, a product, that interferes with growth.
MM: Okay, so it’s part of a normal developmental process in which genes turn on and off.
DR: Right. This is sort of stuck, like getting your accelerator stuck.
MM: Like one of the old record players when the needle got stuck and wouldn’t move on. That’s interesting. I remember that when you were talking about the pygmies, you found that they were growth hormone resistant.
DR: Right.
MM: And is that true of many people with different forms of dysplasia as well?
DR: Well, no. They’re not resistant, they’re just not responsive because that’s not what their basic defect is. If you give anyone growth hormone, they’ll grow somewhat. We did studies on the effects of growth hormone in all skeletal dysplasias and found they do increase their growth in the first year, or maybe two, and then [the growth rates] fall off and you don’t really know whether you end up with any more at the end of growth than if you just left them alone.
MM: Okay. So, it’s sort of inconclusive. Well, let’s see. One of the organizations that’s been very prominent in funding various types of what we used to call birth defects is the March of Dimes. I believe they gave you a lifetime achievement award not too long ago.
DR: Yes. I was fortunate to receive the Colonel Harlan Sanders Lifetime Achievement Award [152] in genetics from the March of Dimes. They’re a great organization. I’ve been involved with them on their advisory boards for thirty years and [have been] on and off various grant review committees and such. They really have done an excellent job in stimulating research of birth defects.
MM: I don’t know how much you can tell me about this. I have a long interest in the March of Dimes because a lot of my early research was on polio. And they had this kind of identity shift, after polio vaccines were developed, and decided to choose birth defects as the main thrust of the organization. And after that, I just sort of lost track of them. Okay, so, I’m just wondering if you have had some sense through your long association with them of how they frame their mission. I mean, birth defects is a pretty global charge, and unlike the government, they have to raise all their money.
DR: Right. Well, they’ve shifted over the years. It was really to prevent birth defects. I think that during the mid-seventies when prenatal diagnosis became prominent, they got a little gun shy at publicity of stimulating abortion, if you will, and shied away from that aspect of it for a while. They became much more interested in perinatal causes and perinatal medicine as a means of preventing birth defects due to prematurity. And that’s been a major part of their mission right now is the prenatal aspects of care. However, they’re still actively involved in funding genetics research in a variety of different areas. So they’re really doing both.
And their research grants are not huge, but they are often stimulatory in getting people started, stimulating research through the Basil O’Connor program [153] to fund young investigators. I think it’s been excellent and which will lead to larger NIH grants in the future.
MM: Let’s hope so. Aside from your own work with the skeletal dysplasias, and certainly, I guess, aside from the human genome, is there particular research that’s going on in the country at the present time or in the last few years that you think is really interesting?
DR: Well, I think that a lot of the research leading to neurogenetics and neurobiology has been fascinating. To see how one can eventually link up biology and psychology, I think, would be fascinating. We’ll really be there one day, I think, in understanding what makes people tick, if you will, and how various chemical changes modify mood and feeling.
One of the things that is intriguing me is, What is it that makes you interested in one thing and somebody else interested in another? Is it all environmental and exposure, or is it something that your sort of half certain innate understanding and qualities, too.
MM: It is fascinating, isn’t it? But as, you know, your own work would find, there must be a variety of genetic factors.
DR: Oh, yes, multiple ones. But I think that we’re going to learn a lot more about neural functioning.
MM: What about genetic therapy? I mean, obviously, in some instances, we seem to be already flirting with the possibilities, but —
DR: For gene therapy?
MM: Yes. But I have a sense this is still fifteen years or so down the road before we can seriously consider gene therapy.
DR: Well, gene therapy, I think, is something that is here, certainly in the bubble baby syndrome, for example. That works. The major reason it does is that you can take out somebody’s bone marrow, put the gene in, put their own marrow back, and make it work for two reasons: one, you’re not using any artificial vector. You’re just putting it right into the person’s marrow. And that person’s marrow is not going to reject itself, which is one of the major things. And it’s producing a substance that normally is produced in the marrow that circulates through the body. For most of the conditions, what you’re going to have to do is get the gene to the right place, so you need a smart bomb. And then you’ve got to turn it on and off at the right times, so you need a smart detonator. And those are the two areas that are going to have to be conquered.
I think that once the technology of this, of finding the right vector and getting it in the right place and being able to turn it on and off appropriately, then having all of these genes available through the human genome project will make the next step a snap, in terms of that. So I think we’re building up to a big technological advance that’s necessary. Then all our knowledge will be able to be taken advantage of.
MM: Okay, we’re really looking – so the breakthrough will probably come in transport technology and the development of radio-employing mechanisms?
DR: Right.
MM: What do you think your own contribution has been to the field?
DR: My own contribution? Oh, I think I made some contributions in terms of my own individual research. I think I made contributions to people I’ve loved, made contributions in training young people, and developing the training programs have been extremely important. I’ve enjoyed producing a book and making it available to people. And what I really enjoy is putting people together and making these organizations work and making genetics a true specialty, which I’ve been fortunate to play a part in. That is, being able to really bring people together and make things happen has been very satisfying.
MM: It clearly is, I think. It comes across that way in what you’ve been saying. Well, I’m sort of at the end of my questions, although I’m sure I could think of some more if I gave it a little bit more time. Thank you very much. It’s been a real pleasure doing this interview.
DR: Well, thank you. It’s been great. As I say, it’s like going to your psychiatrist. (chuckles) I’ll be curious to see how this turns out.
MM: Me, too. We’re concluding and it’s 2:45 pm.
END OF INTERVIEW
ENDNOTES
[141] The venture described by Dr. Rimoin is not connected with the company currently known as GenoMed, based in St. Louis.
[142] Aldons (Jake) Lusis, PhD, is Professor of Medicine and of Microbiology, Immunology, and Molecular Genetics at UCLA. His research interests concern the analysis of complex genetic diseases, resulting from the interaction of multiple genetic and environmental factors.
[143] Nathan Fischel-Ghodsian, MD, is Professor of Pediatrics at UCLA and Director of Molecular Hematology at Cedars-Sinai Medical Center. His research concentrates on the relationships between molecular genetic changes and the clinical phenotype.
[144] Abbott Laboratories, founded as the Abbott Alkaloidal Company in 1900, is today a global manufacturer and distributor of pharmaceuticals, nutrional supplements and medical technologies. Its headquarters are in suburban Chicago.
[145] The Hastings Center is an independent, nonpartisan and nonprofit institute, founded in 1969 and located in Garrison, New York, which conducts and sponsors research on fundamental ethical questions in medicine and healthcare, such as palliative care, decision making at the end of life, setting health policy and priorities, and new technologies. For more information, see http://www.thehastingscenter.org.
[146] Limb lengthening is a procedure used to correct discrepancies in limb length, such as between two extremities, or to lengthen the limbs of congenital little people, or of those who have suffered traumatic injuries or cancerous erosion. The procedure is now often called distraction osteogenesis. The most common procedure, developed by Russian surgeon Gavril Ilizarov (1921-1992) in 1951 is extremely painful and carries a high risk of scarring and infection, and several new procedures, such as Vilarrubias’. are still (2008) being evaluated.
[147] Burr holes are extremely small openings made in bone (usually in the skull) with a surgical drill.
[148] Operating subperiosteally refers to surgical work through the dense periosteal membrane which covers bones along their entire lengths (except at the joints).
[149] Swayback is a common term for a medical condition characterized by an extreme inward curvature of the spine.
[150] Paraplegia is defined as impairment of the motor and sensory functions of both lower extremities. It may be congenital, or result from an acute spinal cord injury, from cord compression due to a tumor or vertebral fracture, or from a neurological disorder such as multiple sclerosis. Most paraplegic patients require an assistive device such as a wheelchair, but some retain the ability to walk.
[151] A cochlear transplant is an artificial device implanted in the ear to stimulate nerve endings and to improve hearing in a person who is profoundly deaf or has severe hearing loss.
[152] Awarded by the March of Dimes Foundation, the Colonel Harland Sanders Award honors pioneers and leaders in clinical genetics. It was funded by the founder of the Kentucky Fried Chicken company, Harland D. Sanders (1890-1980), in 1986.
[153] The Basil O’Connor Starter Scholar Research Award is awarded by the March of Dimes to promising young scientists embarking on independent research careers. The Award is named after Basil O’Connor (1892-1972), co-founder of the March of Dimes/National Foundation and president of the organization from 1938 until his death.